Cobalt oxide nanoparticles
Though the amount of research on cobalt oxide nanoparticles is still relatively new, researchers have found that these particles have great potential for us in chemical and industrial areas.
Characteristics
Cobalt oxide nanoparticles appear white in color and are magnetic p-type semiconductors. It is interesting to note that the particles will convert into cobalt metal upon heating at 900 °C. Even though cobalt oxide nanoparticles have many beneficial uses, the substance is still toxic to humans and also aquatic life. It is harmful when swallowed and also acts as a skin irritant.
Chemical Properties[1]
Chemical Symbol | Co3O4 |
CAS Number | 1308-06-1 |
Group | Cobalt 9 Oxygen 16 |
Electronic Configuration | Cobalt [Ar] 3d74s2 Oxygen [He] 2s22p4 |
Element | Content (%) |
Cobalt | 73.4 |
Oxygen | 26.57 |
Physical Properties
Properties | Metric |
Density | 6.11 g/cm3 |
Molar Mass | 74.93 g/mol |
Thermal Properties
Melting Point | 895 °C |
Boiling Point | 900 °C |
Applications
Although they have been observed to readily enter cells and may possibly be exploited for hyperthermic treatment, gene therapy and drug delivery, a protective and efficient molecular shield for the nanoparticles still needs to be developed.[2] Cobalt nanoparticles are more commonly applied to devices for energy storage.
Lithium-ion Battery
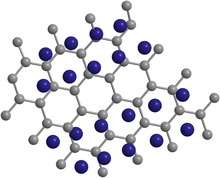
Cobalt oxide nanoparticles are being investigated in optimizing high performance in lithium-ion batteries. This is due to their high surface-to-volume ratio and short path length for lithium cation transport, as opposed to nickel oxide and iron oxides. Some proposals include Co3O4 nanoparticles anchored on graphene as a source of possible lithium battery anodes.[3] This is synthesized by first forming Co(OH)2 on the sheet from Co2+ and NH3H2O, which is then heated to 450 °C for two hours to yield the cobalt oxide/graphene composite. The advantageous combination of the graphene sheet’s flexibility and its strong interaction with Co3O4 nanoparticles prevent volume expansion/contraction and aggregation of Co3O4 during lithium charge and discharge processes.
Porous Co3O4 nanotubes are another good lithium battery anode candidate due to their higher surface-to-volume ratio than other one-dimensional nanostructures.[4] These different types engineered nanostructures exhibited high reversible capacity and good cycle life. Diphenylalanine/cobalt oxide hybrid nanowires have a potential application as anodes for Li-ion batteries as well, also enhancing the specific capacity as an electrode.[5]
Gas Sensor
The p-type Co3O4 hollow nanospheres have performed well as a gas sensor in sensing toluene, acetone, and other organic vapors with rapid response and high sensitivity to very low concentrations at low temperature. This serves to potentially monitor organic flammable and toxic gases.[6] Co3O4 nanoparticles prepared on single-walled carbon nanotubes (SWCNTs) are being investigated in sensing NOx and H2 gases. This takes advantage of Co3O4’s active reactivity with the gases, as well as the electrical continuum formed within the Co3O4 nanoparticle/SWCNT composite. With NOx, the resistance of the sensor decreases because of an increase in the hole density in the composite. This is because both the Co3O4 nanoparticles and carbon nanotubes are p-type semiconductors and NOx is electron-accepting. Thus, the adsorbed gas molecules continue to extract electrons from them. Oppositely, the resistance of the sensor increases for H2 because it is electron donating. “The composite shows a highly enhanced response of ~200 % upon exposure to 4 % H2 diluted in air compared with pure SWCNTs and Co3O4 film” at room temperature.[7]
Synthesis
Hydrothermal Synthesis
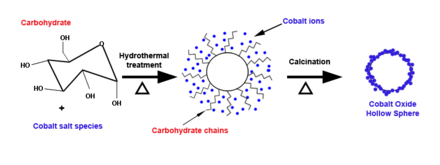
Hydrothermal synthesis of metal oxide hollow spheres takes place in an autoclave and proceeds through a one-pot synthesis to increase yield while reducing time and resources. To initiate the procedure, carbohydrates and metal salts are dissolved in water at 100-200 °C.[8] Carbon spheres are produced with metal ions integrated into the hydrophobic shell. Calcination allows for removal of the carbon from the product and results in hollow metal oxide spheres. Surface area and thickness of the shell can be manipulated by varying the carbohydrate to metal salt concentration.[9] The completion time for the procedure varies from hours to days.[10] Hydrothermal synthesis is generally simple and being able to control size and shape is beneficial for specificity. However, the drawback of this approach is a smaller yield compared to other methods. The pH of the reaction medium, temperature, pressure, and cations used in the solution all play a role in determining the nature of the solid. This approach allows for the production of a wide range of shapes and sizes for metal oxide hollow spheres.
Solvent Free Thermal Synthesis
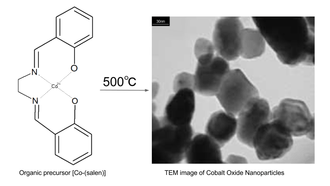
Cobalt oxide (Co3O4) nanoparticles are generally synthesized at low temperature; However, the tendency of Co3O4 to grow into complex microstructure made the addition of organic surfactant necessary. Organic surfactant is both expensive and pollutant. Using organometallic molecular as a precursor to synthesize Co3O4 under high temperature can ensure purity, composition, phase purity, and microstructure.[11] Using organometallic chemistry, Co3O4 is synthesized by heating 0.01 mol of [bis(salicylaldehyde)ethylenediiminecobalt(II], known as [ Co-(salen) ], to 500 °C in the air. The synthesis of the organometallic molecular precursor [Co-(salen)] is prepared by first making the bis(salicylaldehyde)ethylenediimine crystal. By dissolving salicylaldehyde in methanol with drops of 1,2- ethylenediamine, 92% of bis(salicylaldehyde)ethylenediimine crystal could be purified via reflux and filtration. [Bis(salicylaldehyde)ethylenediirninccobalt(II)] is then prepared by adding cobalt(II) acetate tetrahydrate in propanol and warmed to 50 °C under nitrogen atmosphere, followed by adding bis(salicylaldehyde)ethylenediimine and purification through reflux and recrystallization.[12]
References
- ↑ "Cobalt Oxide (Co3O4) Nanoparticles – Properties, Applications". Retrieved 20 March 2015.
- ↑ Papis, Elena; Rossi, Federica; Raspanti, Mario; Dalle-Donne, Isabella; Colombo, Graziano; Milzani, Aldo; Bernardini, Giovanni; Gornati, Rosalba (September 2009). "Engineered cobalt oxide nanoparticles readily enter cells". Toxicology Letters. 189 (3): 253–259. doi:10.1016/j.toxlet.2009.06.851.
- ↑ Wu, Zhong-Shuai; Ren, Wencai; Wen, Lei; Gao, Libo; Zhao, Jinping; Chen, Zongping; Zhou, Guangmin; Li, Feng; Cheng, Hui-Ming (22 June 2010). "Graphene Anchored with Co O Nanoparticles as Anode of Lithium Ion Batteries with Enhanced Reversible Capacity and Cyclic Performance". ACS Nano. 4 (6): 3187–3194. doi:10.1021/nn100740x.
- ↑ Du, N.; Zhang, H.; Chen, B. D.; Wu, J. B.; Ma, X. Y.; Liu, Z. H.; Zhang, Y. Q.; Yang, D. R.; Huang, X. H.; Tu, J. P. (17 December 2007). "Porous Co3O4 Nanotubes Derived From Co4(CO)12 Clusters on Carbon Nanotube Templates: A Highly Efficient Material For Li-Battery Applications". Advanced Materials. 19 (24): 4505–4509. doi:10.1002/adma.200602513.
- ↑ Ryu, Jungki; Kim, Sung-Wook; Kang, Kisuk; Park, Chan Beum (26 January 2010). "Synthesis of Diphenylalanine/Cobalt Oxide Hybrid Nanowires and Their Application to Energy Storage". ACS Nano. 4 (1): 159–164. doi:10.1021/nn901156w.
- ↑ Park, Jinsoo; Shen, Xiaoping; Wang, Guoxiu (March 2009). "Solvothermal synthesis and gas-sensing performance of Co3O4 hollow nanospheres". Sensors and Actuators B: Chemical. 136 (2): 494–498. doi:10.1016/j.snb.2008.11.041.
- ↑ Li, Wei; Jung, Hyuck; Hoa, Nguyen Duc; Kim, Dojin; Hong, Soon-Ku; Kim, Hyojin (September 2010). "Nanocomposite of cobalt oxide nanocrystals and single-walled carbon nanotubes for a gas sensor application". Sensors and Actuators B: Chemical. 150 (1): 160–166. doi:10.1016/j.snb.2010.07.023.
- ↑ Whittingham, M Stanley (April 1996). "Hydrothermal synthesis of transition metal oxides under mild conditions". Current Opinion in Solid State and Materials Science. 1 (2): 227–232. doi:10.1016/S1359-0286(96)80089-1.
- ↑ Titirici, Maria-Magdalena; Antonietti, Markus; Thomas, Arne (August 2006). "A Generalized Synthesis of Metal Oxide Hollow Spheres Using a Hydrothermal Approach". Chemistry of Materials. 18 (16): 3808–3812. doi:10.1021/cm052768u.
- ↑ Lu, An-Hui; Salabas, E. L.; Schüth, Ferdi (12 February 2007). "Magnetic Nanoparticles: Synthesis, Protection, Functionalization, and Application". Angewandte Chemie International Edition. 46 (8): 1222–1244. doi:10.1002/anie.200602866.
- ↑ Sharma, J.K.; Srivastava, Pratibha; Singh, Gurdip; Akhtar, M. Shaheer; Ameen, S. (March 2015). "Green synthesis of Co3O4 nanoparticles and their applications in thermal decomposition of ammonium perchlorate and dye-sensitized solar cells". Materials Science and Engineering: B. 193: 181–188. doi:10.1016/j.mseb.2014.12.012.
- ↑ Salavati-Niasari, Masoud; Khansari, Afsaneh (April 2014). "Synthesis and characterization of Co3O4 nanoparticles by a simple method". Comptes Rendus Chimie. 17 (4): 352–358. doi:10.1016/j.crci.2013.01.023.