Oddo–Harkins rule
The Oddo–Harkins rule holds that elements with an even atomic number (such as carbon) are more common than elements with an odd atomic number (such as nitrogen). This effect on the abundance of the chemical elements was first reported by Giuseppe Oddo[1] in 1914 and William Draper Harkins[2] in 1917.[3]
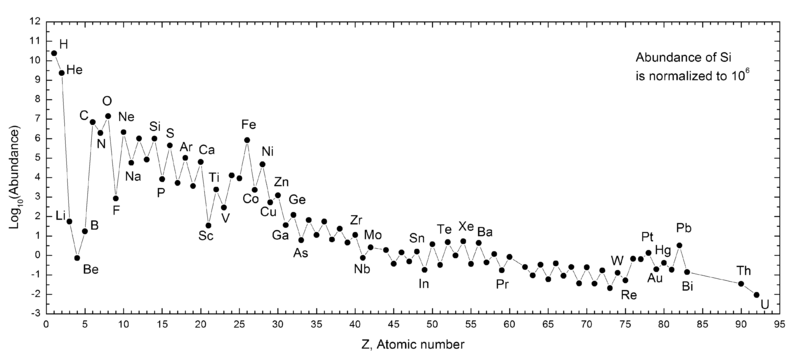
Definition
All atoms bigger than hydrogen are made in stars when gravity, heat and pressure cooperate to persuade protons and neutrons to clump together in a process called nuclear fusion or in the process of a supernova. Later, after the clumps have had a chance to cool off and accumulate electrons for their shells, each is the nucleus of a new atom. The number of protons that the nucleus ends up with is the atomic number, and that is also the normal number of electrons around it, making the atom electrically neutral. The number of neutrons in an atom varies.
This rule argues that elements with odd atomic numbers have one unpaired proton and are more likely to capture another, thus increasing their atomic number. It is possible that in elements with even atomic numbers, partons are paired, with each member of the pair balancing the spin of the other; even parity thusly enhances nucleon stability.
Exceptions to the rule
This postulate, however, is completely untrue for the universe's most abundant, and simplest element from the periodic table of elements: hydrogen, with an atomic number of 1. Perhaps this is simply because of the fact that, in its ionized form, a hydrogen atom becomes a single proton, of which is theorized to have been one of the first major conglomerates of quarks during the initial second of the Universe's inflation period, following the Big Bang. In this period, when inflation of the universe had brought it from an infinitesimal point to about the size of a modern galaxy, temperatures in the particle soup fell from over a trillion degrees to several million degrees.
This period allowed for the fusion of single protons and deuterium nuclei to form helium and lithium nuclei but remained brief and far too short for every H+ ion to be reconstituted into heavier elements. In this case, helium, atomic number 2, remains the even numbered counterpart to hydrogen. Thus, neutral hydrogen - or hydrogen paired with an electron, the only stable lepton - constituted the vast majority of the remaining unannihilated portions of matter following the conclusion of inflation.
Another notable exception to the rule is beryllium, which, even with an even atomic number (4) is more rare than the odd number elements to either side of it (lithium and boron). This discrepancy results from the fact that most of the universe's lithium, beryllium, and boron are made by cosmic ray spallation, not ordinary stellar nucleosynthesis, and beryllium has only one stable isotope, causing it to lag in abundance with regard to its chemical element neighbors, which each have two stable isotopes.
Relationship to fusion
In a natural sense, the pattern arises right after the runaway fusion in a dying supermassive star occurs, in which a given mass of the various even and odd numbered elements are formed by a slightly greater mass of the elements hydrogen and helium; wherein the mass of elements is exploded outward from the star's outer-interior to join the rest of the galaxy's interstellar medium. In this case, the postulate gets revised to include the increased probability of relevance on a universal scale as the atomic mass of the element is increased, by factoring in the decrease in energy output, and thus feasibility, of fusing subsequently larger atomic nuclei.
Basically, what this means is that when fusion occurs with larger and larger nuclei, the energy input becomes increasingly larger and the energy output becomes increasingly smaller; the point at which these two potentials meet on the periodic table of elements is somewhere around the elements iron, atomic number 26, and nickel, atomic number 28. And so from here on fusion becomes exponentially more and more unachievable making the probability of finding discrepancies in the Oddo–Harkins rule more and more doubtful.
See also
- Atomic number
- Hydrogen
- Cosmic inflation
- List of elements by stability of isotopes
- Nuclear fusion
- Nuclear chemistry
References
- ↑ Oddo, Giuseppe (1914). "Die Molekularstruktur der radioaktiven Atome". Zeitschrift für anorganische Chemie. 87: 253. doi:10.1002/zaac.19140870118.
- ↑ Harkins, William D. (1917). "The Evolution of the Elements and the Stability of Complex Atoms". Journal of the American Chemical Society. 39 (5): 856. doi:10.1021/ja02250a002.
- ↑ North, John (2008). Cosmos an illustrated history of astronomy and cosmology (Rev. and updated ed.). Univ. of Chicago Press. p. 602. ISBN 978-0-226-59441-5.