Ore resources on Mars
Mars may contain ores that would be very useful to potential colonists.[1] The abundance of volcanic features together with widespread cratering are strong evidence for a variety of ores. While nothing may be found on Mars that would justify the high cost of transport to Earth, the more necessary ores future colonists can obtain from Mars, the easier it would be to build colonies on the Red Planet.[2]
How deposits are made
Ore deposits are produced with the help of large amounts of heat. On Mars, heat can come from molten rock moving under the ground and from crater impacts. Liquid rock under the ground is called magma. When magma sits in underground chambers, slowly cooling over thousands of years, heavier elements sink. These elements, including copper, chromium, iron, and nickel become concentrated at the bottom.[3] When magma is hot, many elements are free to move. As cooling proceeds, the elements bind with each other to form chemical compounds or minerals. Because some elements do not bond easily to form minerals, they exist freely after nearly all the other elements have bonded into compounds or minerals. The remaining elements are called incompatible elements.[4] Some of them are quite useful to humans. Some examples include niobium, a metal used in producing superconductors and specialty steels, lanthanum and neodymium, and europium for television monitors and energy-efficient LED light bulbs. [5] After the mass of magma has cooled and has mostly frozen or crystallized into a solid, a small amount of liquid rock remains. This liquid bears important substances such as lead, silver, tin, bismuth, and antimony.[6] Sometimes minerals in the magma chamber are so hot that they occupy a gaseous state. Others are mixed with water and sulfur in aqueous solutions. The gases and mineral-rich solutions eventually work their way into cracks and become useful mineral veins. Ore minerals, including the incompatible elements, remain dissolved in the hot solution, then crystallize out when the solution cools.[7] Deposits created by means of these hot solutions are called hydrothermal deposits. Some of the world's most significant deposits of gold, silver, lead, mercury, zinc, and tungsten started out this way.[8][9][10] Nearly all the mines in the northern Black Hills of South Dakota came to be because of hot water deposits of minerals.[11] Cracks often form when a mass of magma cools because magma contracts when it cools. Cracks occur both in the frozen magma mass and in the surrounding rocks, so ore is deposited in any kind of the rock that happens to be nearby, but the ore minerals first had to be concentrated by way of a hot, molten mass of magma.[12]
Molten rock on Mars
The presence of many huge volcanoes on Mars shows that large areas were very hot in the past. Olympus Mons is the largest volcano in the solar system; Ceraunius Tholus, one of its smaller volcanoes, nears the height of Earth's Mt. Everest.
-
Olympus Mons Region showing several large volcanoes.
-
Lower volcano is Ceraunius Tholus and upper volcano is Uranius Tholus as seen by Mars Global Surveyor Mars Orbiter Camera. Ceraunius Tholus is about as high as Earth's Mount Everest.
-
Lava flow, as seen by THEMIS. Note the shape of the edges
What's more, there is strong evidence for much more widespread sources of heat in the form of dikes, which indicate that magma traveled under the ground. Dikes take the shape of walls and cut across rock layers.[13] In some cases dikes on Mars have become exposed by erosion.
-
Possible dike in Syrtis Major quadrangleas seen by HiRISE under the HiWish program.
-
Dikes in Arabia, as seen by HiRISE, under the HiWish program. These straight features may indicate where valuable ore deposits may be found by future colonists. Scale bar is 500 meters.
-
Possible dike in Thaumasia quadrangle, as seen by HiRISE under HiWish program. Dikes may have deposited valuable minerals.
-
Possible dikes in Oxia Palus quadrangle, as seen by HiRISE under HiWish program.
-
Possible dike, as seen by HiRISE under HiWish program Image located in Iapygia quadrangle.
-
Straight ridges may be dikes in which liquid rock once flowed. Image is of Huo Hsing Vallis in Syrtis Major, as seen by THEMIS.
-
Dikes as seen by HiRISE under the HiWish program. Image in Nilosyrtis region, in Casius quadrangle.
-
Dikes near Spanish Peaks, Colorado. Dikes like these are common on Mars
Large areas of Mars contain troughs, called fossa, which are classified as grabens by geologists. They stretch thousands of miles out from volcanoes.[14] It is believed that dikes helped with the formation of grabens.[15][16][17] Many, maybe most, of the grabens had dikes under them. One would expect dikes and other igneous intrusions on Mars because geologists believe that the amount of liquid rock that moved under the ground is more than what we see on the top in the form of volcanoes and lava flows.[18] On Earth, vast volcanic landscapes are called "Large Igenous Provinces" (LIP's); such places are sources of nickel, copper, titanium, iron, platinum, palladium, and chromium.[2][19] Mars's Tharsis region, which contains a group of giant volcanoes, is considered to be an LIP.
-
Graben in Memnonia Fossae, as seen by HiRISE. This graben is believed to be the result of magmatic dikes rather than regional tectonic stretching. The scale bar is 1000 metres long.
-
Cerberus Fossae in the Elysium quadrangle, as seen by HiRISE
Heat from impacts
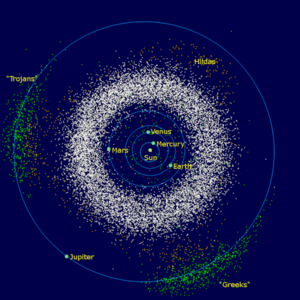
Besides heat generated by molten rock, Mars has had much heat produced when asteroids impacted its surface making giant craters. The area around a large impact may take hundreds of thousands of years to cool.[2]

During that time, ice in the ground will melt, heat, dissolve minerals, then deposit them in cracks or faults that were produced with the impact. Studies on the earth have documented that cracks are produced and that secondary minerals veins are filled in the cracks.[20] [21] [22] Images from satellites orbiting Mars have detected cracks near impact craters.[23] Areas of aqueous and low-grade thermal alteration have been found by the Opportunity Rover on the rim of Endeavour crater.[24] These are found near joints and fractures that allowed deep fluid circulation which caused chemical and thermal alteration of the rocks. So, the area around martian craters may contain a variety of minerals that were produced as an indirect result of an impact.[25]
Strong evidence for hydrothermalism was reported by a team of researchers studying Auki Crater. This crater contains ridges that may have been produced after fractures formed with an impact. Using instruments on the Mars Reconnaissance Orbiter they found the minerals smectite, silica, zeolite, serpentine, carbonate, and chorite that are common in impact-induced hydrothermal systems on Earth.[26] [27] [28] [29] [30] [31] Other evidence of post-impact hydrothermal systems on Mars from other scientists who studied other Martian craters.[32] [33] [34]
-
Wide view of Auki Crater, as seen by CTX
-
Close view of central portion of Auki Crater, as seen by HiRISE Arrow indicates ridges. Sand dunes are present near the top of the image.
-
Close view of ridges from previous HiRISE image Arrow indicates an "X" shaped ridge.
-
Close view of central section of Auki Crater showing ridges with arrow Image is an enlargement of a previous HiRISE image.
The surface of Mars contains abundant evidence of a wetter climate in the past along with ice frozen in the ground. NASA's Mars Odyssey actually measured the distribution of ice from orbit with a gamma ray spectrometer.[35] So, in the past, much water could have been available to circulate in cracks and deposit new minerals. This process, called hydrothermal alteration has been found in a meteorite from Mars. Research, published in February 2011, detailed the discovery of clay minerals, serpentine, and carbonate in the veins of a Nakhlite martian meteorite.[36][37] The Phoenix lander, whose rocket engine blast actually exposed a layer of ice, watched ice melt (the ice disappeared by sublimation).[38][39]
Because 30% of the roughly 180 impact craters on Earth contain minerals or oil and gas, it seems that the cratering promotes the development of natural resources [40]
-
Hellas Basin Area topography. This is one of the impacts that would have taken many thousands of years to cool. A great deal of minerals could have been deposited while this area was cooling.
-
Topography of the Argyre Basin, the major feature in the Argyre quadrangle. This large impact crater would have also taken many thousands of years to cool.
Direct evidence for useful materials

It has for some time been accepted by the scientific community that a group of meteorites came from Mars. As such, they represent actual samples of the planet and have been analyzed on Earth by the best equipment available. In these meteorites, called SNCs, many important elements have been detected. Magnesium, Aluminium, Titanium, Iron, and Chromium are relatively common in them. In addition, lithium, cobalt, nickel, copper, zinc, niobium, molybdenum, lanthanum, europium, tungsten, and gold have been found in trace amounts. It is quite possible that in some places these materials may be concentrated enough to be mined.[41]
The Mars landers Viking I, Viking II, Pathfinder, Opportunity Rover, and Spirit Rover identified aluminium, iron, magnesium, and titanium in the Martian soil.[42] Opportunity found small structures, named "blueberries" which were found to be rich in hematite, a major ore of iron.[43] These blueberries could easily be gathered up and reduced to metallic iron that could be used to make steel.
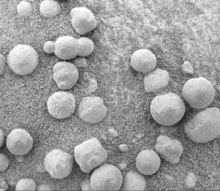
In addition, both Spirit and Opportunity Rovers found nickel-iron meteorites sitting on the surface of Mars.[44][45] These could also be used to produce steel.[46]
In December 2011, Opportunity Rover discovered a vein of gypsum sticking out of the soil. Tests confirmed that it contained calcium, sulfur, and water. The mineral gypsum is the best match for the data. It likely formed from mineral-rich water moving through a crack in the rock. The vein, called "Homestake," is in Mars' Meridiani plain. Homestake is in a zone where the sulfate-rich sedimentary bedrock of the plains meets older, volcanic bedrock exposed at the rim of Endeavour crater.[47]
-
Heat Shield Rock was the first meteorite ever identified on another planet. It is 93% iron.

-
Viking lander took this picture of the Martian surface and analyzed the soil.
Dark sand dunes are common on the surface of Mars. Their dark tone is due to the volcanic rock called basalt. The basalt dunes are believed to contain the minerals chromite, magnetite, and ilmenite.[48] Since the wind has gathered them together, they do not even have to be mined, merely scooped up.[49] These minerals could supply future colonists with chromium, iron, and titanium.
-
Dark dunes (probably basalt) which form a dark spot in Noachis quadrangle. Picture from Mars Global Surveyor.
-
Wide view of dunes in Noachis, as seen by HiRISE.
-
Close-up View of dunes in previous image, as seen by HiRISE. Note how sand barely covers some boulders.
Future detection for ores on Mars
Theoretically, ore resources exist on Mars.[49] Moreover, sensitive equipment can predict where to look for them, such as around craters and near volcanic regions. As more images are gathered, more information will be gathered which will help to better map the locations of smaller structures, such as dikes, that indicate intrusive (under the surface) igneous activity. Later, flying unmanned craft with gravity and magnetic measuring devices will be able to determine the exact locations of mineral deposits. These devices were employed in Afghanistan by American scientists to discover deposits of iron, copper, niobium, lithium and gold.[50]
See also
References
- ↑ Cordell, B. 1984. A Preliminary Assessment of Martian Natural Resource Potential. The Case For Mars II.
- 1 2 3 Larry O'Hanlon (February 22, 2010). "Mining Mars? Where's the Ore?". Discovery News.
- ↑ Namowitx, S. and D. Stone. 1975. Earth Science: The World We Live In. American Book Company. NY, NY.
- ↑ http://home.wlu.edu/~kuehns/geo311/f09/igneous6.pdf
- ↑ http://www.livescience.com/technology/Rare-Earth-Elements-100614.html
- ↑ Sorrell, C. 1973. Rocks and Minerals. Golden Press. NY, NY.
- ↑ http://www.indiana.edu/~sierra/papers/2003/Patterson.html
- ↑ http://nevada-outback-gems.com/prospect/gold_specimen/California_quartz_veins.htm
- ↑ http://www.springerlink.com/content/r87p498m051rm18t/
- ↑ http://www.mirandagold.com/s/CoalCanyon.asp
- ↑ ISBN 0-87842-338-9
- ↑ Pirajno, F. 2004. Metallogeny in the Capricorn Orogen, Western Australia, the result of multiple ore-forming processes. Precambrian Research: 128. 411-439
- ↑ http://www.mantleplumes.org/GiantRadDykeSwarms.html
- ↑ Head, J. et al. 2006. The Huygens-Hellas giant dike system on Mars: Implications for Late Noachian-Early Hesperian volcanic resurfacing and climate evolution. Geology: 34. 285-288.
- ↑ Goudy, C. and R. Schultz. 2005. Dike intrusions beneath grabens south of Arsia Mons, Mars. Geophysical Research Letters: 32. L05201
- ↑ Mege, D. et al. 2003. Volcanic rifting at Martian grabens. Journal of Geophysical Research: 108.
- ↑ Wilson, L. and J. Head. 2002. Tharsis-radial graben systems as the surface manifestation of plume-related dike intrusion complexes: Models and implications. Journal of Geophysical Research: 107.
- ↑ Crisp, J. 1984. Rates of magma emplacement and volcanic output. J. Volcanlo. Geotherm. Res: 20. 177-211.
- ↑ Ernst, R. 2007. large Igneous Provinces in Canada Through Time and Their Metallogenic Potential. Mineral Deposits of Canada: A Synthesis of Major Depotit-Types, District metallogeny, the Evolution of Geological Provinces, and Exporation Methods: Geological Association of Canada, Mineral Division, Special Publication No. 5. 929-937.
- ↑ Osinski, G, J. Spray, and P. Lee. 2001. Impact-induced hydrothermal activity within the Haughton impact structure, arctic Canada: Generation of a transient, warm, wet oasis. Meteoritics & Planetary Science: 36. 731-745
- ↑ http://www.ingentaconnect.com/content/arizona/maps/2005/00000040/00000012/art00007
- ↑ Pirajno, F. 2000. Ore Deposits and Mantle Plumes. Kluwer Academic Publishers. Dordrecht, The Netherlands
- ↑ Head, J. and J. Mustard. 2006. Breccia Dikes and Crater-Related Faults in Impact Craters on Mars: Erosion and Exposure on the Floor of a 75-km Diameter Crater at the Dichotomy Boundary. Special Issue on Role of Volatiles and Atmospheres on Martian Impact Craters Meteoritics & Planetary Science
- ↑ Arvidson, R., et al. 2015. RECENT RESULTS FROM THE OPPORTUNITY ROVER’S EXPLORATION OF ENDEAVOUR CRATER, MARS. 46th Lunar and Planetary Science Conference. 1118.pdf
- ↑ Crumpler, L., R. Arvidson, W. Farrand, M. Golombek, J. Grant, D. Ming, D. Mittlefehldt, T. Parker. 2015. OPPORTUNITY IN SITU GEOLOGIC CONTEXT OF AQUEOUS ALTERATION ALONG OFFSETS IN THE RIM OF ENDEAVOUR CRATER. 46th Lunar and Planetary Science Conference. 2209.pdf
- ↑ Carrozzo, F. et al. 2017. Geology and mineralogy of the Auki Crater, Tyrrhena Terra, Mars: A possible post impact-induced hydrothermal system. 281: 228-239
- ↑ Loizeau, D. et al. 2012. Characterization of hydrated silicate-bearing outcrops in tyrrhena Terra, Mars: implications to the alteration history of Mars. Icarus: 219, 476-497.
- ↑ Naumov, M. 2005. Principal features of impact-generated hydrothermal circulation systems: mineralogical and geochemical evidence. Geofluids: 5, 165-184.
- ↑ Ehlmann, B., et al. 2011. Evidence for low-grade metamorphism, hydrothermal alteration, and diagenesis on Mars from phyllosilicate mineral assemblages. Clays Clay Miner: 59, 359-377.
- ↑ Osinski, G. et al. 2013. Impact-generated hydrothermal systems on Earth and Mars. Icarus: 224, 347-363.
- ↑ Schwenzer, S., D. Kring. 2013. Alteration minerals in impact-generated hydrothermal systems – Exploring host rock variability. Icarus: 226, 487-496.
- ↑ Marzo, G., et al. 2010. Evidence for hesperian impact-induced hydrothermalism on Mars. Icarus: 667-683.
- ↑ Mangold, N., et al. 2012. Hydrothermal alteration in a late hesperian impact crater on Mars. 43th Lunar and Planetary Science. #1209.
- ↑ Tornabene, L., et al. 2009. Parautochthonous megabreccias and possible evidence of impact-induced hydrothermal alteration in holden crater, Mars. 40th LPSC. #1766.
- ↑ http://mars.jpl.nasa.nasa.gov/odyssey/newsroom/pressreleases/20020528a.html
- ↑ http://www.spaceref.com/news/viewpr.html?pid=32629
- ↑ H. G. Changela and J. C. Bridges. Alteration assemblages in the nakhlites: Variation with depth on Mars. Meteoritics & Planetary Science, 2011 45(12):1847-1867 doi:10.1111/j.1945-5100.2010.01123.x
- ↑ Rayl, A. J. S. (2008-06-21). "Phoenix Scientists Confirm Water-Ice on Mars". The Planetary Society web site. Planetary Society. Retrieved 2008-06-23. External link in
|work=
(help) - ↑ Confirmation of Water on Mars
- ↑ http://www.spaceref.com/news/viewpr.html?pid=37633
- ↑ Hugh H. Kieffer (1992). Mars. University of Arizona Press. ISBN 978-0-8165-1257-7. Retrieved 7 March 2011.
- ↑ Fairen, A. et al. 2009. Nature: 459. 401-404.
- ↑ Squyres, et al. 2004. The Opportunity Rover's Athena science investigation at Meridiani Planum. Science: 306. 1598-1703.
- ↑ Rodionov, D. et al. 2005. An iron-nickel meteorite on Meridiani Planum: observations by MER Opportunity's Mossbauer Spectrometer, European Geosciences Union in Geophysical Research Abstracts: 7. 10242
- ↑ Yen, A., et al. Nickel on Mars: constraints on meteoritic material at the surface. Journal of Geophysical Research-Planets: 111. E12S11
- ↑ Landis, G. 2009. Meteoritic steel as a construction resource on Mars. Acta Astronautica: 64. 183-187.
- ↑ http://www.nasa.gov/home/hqnews/2011/dec/HQ_11-403_Mars_Rover_Gypsum.html
- ↑ Ruzicka, G. et al. 2001. Comparative geochemistry of basalts from the Moon, Earth, HED asteroid, and Mars: implications for the origin of the Moon. Geochimica et Cosmochimica ACTA: 65. 979-997.
- 1 2 West, M. and J. Clarke. 2010. Potential martian mineral resources: Mechanisms and terrestrial analogues. Planetary and Space Science: 58. 574-582.
- ↑ http://nytimes.com/2010/06/14/world/asia/14minerals.html?page watched=2