Oxalate

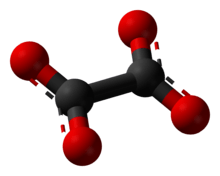
Oxalate (IUPAC: ethanedioate) is the dianion with the formula C
2O2−
4, also written (COO)2−
2. Either name is often used for derivatives, such as salts of oxalic acid, for example sodium oxalate Na2C2O4, or dimethyl oxalate ((CH3)2C2O4). Oxalate also forms coordination compounds where it is sometimes abbreviated as ox.
Many metal ions form insoluble precipitates with oxalate, a prominent example being calcium oxalate, the primary constituent of the most common kind of kidney stones.
Relationship to oxalic acid
The dissociation of protons from oxalic acid proceeds in a stepwise manner as for other polyprotic acids. Loss of a single proton results in the monovalent hydrogenoxalate anion HC
2O−
4. A salt with this anion is sometimes called an acid oxalate, monobasic oxalate, or hydrogen oxalate. The equilibrium constant (Ka) for loss of the first proton is 5.37 × 10−2 (pKa = 1.27). The loss of the second proton, which yields the oxalate ion has an equilibrium constant of 5.25 × 10−5 (pKa = 4.28). These values imply, in solutions with neutral pH, no oxalic acid and only trace amounts of hydrogen oxalate exist.[1] The literature is often unclear on the distinction between H2C2O4, HC
2O−
4, and C
2O2−
4, and the collection of species is referred to as oxalic acid.
Structure
X-ray crystallography of simple oxalate salts show that the oxalate anion may adopt either a planar conformation with D2h molecular symmetry, or a conformation where the O–C–C–O dihedrals approach 90° with approximate D2d symmetry.[2] Specifically, the oxalate moiety adopts the planar, D2h conformation in the solid-state structures of M2C2O4 (M = Li, Na, K).[3][4] However, in structure of Cs2C2O4 the O–C–C–O dihedral angle is 81(1)°.[5][6] Therefore, Cs2C2O4 is more closely approximated by a D2d symmetry structure because the two CO2 planes are staggered. Interestingly, two forms of Rb2C2O4 have been structurally characterized by single-crystal X-ray diffraction; one contains a planar and the other a staggered oxalate.
As the preceding examples indicate that the conformation adopted by the oxalate dianion is dependent upon the size of the alkali metal to which it is bound, some have explored the barrier to rotation about the central C−C bond. The barrier to rotation about this bond was determined computationally to be roughly 2–6 kcal/mol for the free dianion, C
2O2−
4.[7][8][9] Such results are consistent with the interpretation that the central carbon–carbon bond is best regarded as a single bond with only minimal pi interactions between the two CO−
2 units.[2] This barrier to rotation about the C−C bond (which formally corresponds to the difference in energy between the planar and staggered forms) may be attributed to electrostatic interactions as unfavorable O−O repulsion is maximized in the planar form.
Importantly, oxalate is often encountered as a bidentate, chelating ligand, such as in potassium ferrioxalate. When the oxalate chelates to a single metal center, it always adopts the planar conformation.
Occurrence in nature
Oxalate occurs in many plants, where it is synthesized by the incomplete oxidation of carbohydrates.
Oxalate-rich plants include fat hen ("lamb's quarters"), sorrel, and several Oxalis species. The root and/or leaves of rhubarb and buckwheat are high in oxalic acid.[10] Other edible plants that contain significant concentrations of oxalate include, in decreasing order, star fruit (carambola), black pepper, parsley, poppy seed, amaranth, spinach, chard, beets, cocoa, chocolate, most nuts, most berries, fishtail palms, New Zealand spinach (Tetragonia tetragonioides), and beans. Leaves of the tea plant (Camellia sinensis) contain among the greatest measured concentrations of oxalic acid relative to other plants. However, the beverage derived by infusion in hot water typically contains only low to moderate amounts of oxalic acid due to the small mass of leaves used for brewing.
Common high-oxalate foods[11] | ||
---|---|---|
Food item | Serving |
Oxalate Content (mg) |
Beetroot greens, cooked | 1⁄2 cup (unit) | 916 |
Purslane, leaves, cooked | 1⁄2 cup | 910 |
Rhubarb, stewed, no sugar | 1⁄2 cup | 860 |
Spinach, cooked | 1⁄2 cup | 750 |
Beet, cooked | 1⁄2 cup | 675 |
Chard, Swiss, leaves cooked | 1⁄2 cup | 660 |
Rhubarb, canned | 1⁄2 cup | 600 |
Spinach, frozen | 1⁄2 cup | 600 |
Beet, pickled | 1⁄2 cup | 500 |
Poke greens, cooked | 1⁄2 cup | 476 |
Endive, raw | 20 long leaves | 273 |
Cocoa, dry | 1⁄3 cup | 254 |
Dandelion greens, cooked | 1⁄2 cup | 246 |
Okra, cooked | 8–9 pods | 146 |
Sweet potato, cooked | 1⁄2 cup | 141 |
Kale, cooked | 1⁄2 cup | 125 |
Peanuts, raw | 1⁄3 cup ( 1 3⁄4 oz) | 113 |
Turnip greens, cooked | 1⁄2 cup | 110 |
Chocolate, unsweetened | 1 oz | 91 |
Parsnips, diced, cooked | 1⁄2 cup | 81 |
Collard greens, cooked | 1⁄2 cup | 74 |
Pecans, halves, raw | 1⁄3 cup ( 1 1⁄4 oz) | 74 |
Tea, leaves (4-minute infusion) | 1 level tsp in 7 fl oz water | 72 |
Cereal germ, toasted | 1⁄4 cup | 67 |
Gooseberries | 1⁄2 cup | 66 |
Potato, Idaho white, baked | 1 medium | 64 |
Carrots, cooked | 1⁄2 cup | 45 |
Apple, raw with skin | 1 medium | 41 |
Brussels sprouts, cooked | 6–8 medium | 37 |
Strawberries, raw | 1⁄2 cup | 35 |
Celery, raw | 2 stalks | 34 |
Milk chocolate bar | 1 bar (1.02 oz) | 34 |
Raspberries, black, raw | 1⁄2 cup | 33 |
Orange, edible portion | 1 medium | 24 |
Green beans, cooked | 1⁄2 cup | 23 |
Chives, raw, chopped | 1 tablespoon | 19 |
Leeks, raw | 1⁄2 medium | 15 |
Blackberries, raw | 1⁄2 cup | 13 |
Concord grapes | 1⁄2 cup | 13 |
Blueberries, raw | 1⁄2 cup | 11 |
Redcurrants | 1⁄2 cup | 11 |
Apricots, raw | 2 medium | 10 |
Raspberries, red, raw | 1⁄2 cup | 10 |
Broccoli, cooked | 1 large stalk | 6 |
Cranberry juice | 1⁄2 cup (4 oz) | 6 |
Physiological effects
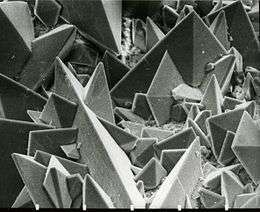
In the body, oxalic acid combines with divalent metallic cations such as calcium (Ca2+) and iron(II) (Fe2+) to form crystals of the corresponding oxalates which are then excreted in urine as minute crystals. These oxalates can form larger kidney stones that can obstruct the kidney tubules. An estimated 80% of kidney stones are formed from calcium oxalate.[12] Those with kidney disorders, gout, rheumatoid arthritis, or certain forms of chronic vulvar pain (vulvodynia) are typically advised to avoid foods high in oxalic acid . Methods to reduce the oxalate content in food are of current interest.[13]
Magnesium (Mg2+) oxalate is 567 times more soluble than calcium oxalate, so the latter is more likely to precipitate out when magnesium levels are low and calcium and oxalate levels are high. Magnesium oxalate is a million times more soluble than mercury oxalate. Oxalate solubility for metals decreases in the order Mg > Ca > Cd > Zn > {Mn, Ni, Fe, Cu} > {As, Sb, Pb} > Hg.
The highly insoluble iron(II) oxalate appears to play a major role in gout, in the nucleation and growth of the otherwise extremely soluble sodium urate. This explains why gout usually appears after age 40, when ferritin levels in blood exceed 1 μg/L. Foods high in oxalate such as dark beers, liver, and yogurt [14] should be avoided by people suffering from, or at risk of gout.[15]
Cadmium catalyzes the transformation of vitamin C into oxalic acid. This can be a problem for people exposed to high levels of cadmium in their diets, in the workplace, or through smoking.
In studies with rats, calcium supplements given along with foods high in oxalic acid can cause calcium oxalate to precipitate in the gut and reduce the levels of oxalate absorbed by the body (by 97% in some cases.)[16][17]
Oxalic acid can also be produced by the metabolism of ethylene glycol ("antifreeze"), glyoxylic acid, or ascorbic acid (vitamin C).[18]
Powdered oxalate is used as a pesticide in beekeeping to combat the bee mite.
Some fungi of the genus Aspergillus produce oxalic acid.[19]
Some preliminary evidence indicates the administration of probiotics can affect oxalic acid excretion rates in a positive manner.[20]
As a ligand
Oxalate, the conjugate base of oxalic acid, is an excellent ligand for metal ions. It usually binds as a bidentate ligand forming a 5-membered MO2C2 ring. An illustrative complex is potassium ferrioxalate, K3[Fe(C2O4)3]. The drug oxaliplatin exhibits improved water solubility relative to older platinum-based drugs, avoiding the dose-limiting side-effect of nephrotoxicity. Oxalic acid and oxalates can be oxidized by permanganate in an autocatalytic reaction. One of the main applications of oxalic acid is a rust-removal, which arises because oxalate forms water-soluble derivatives with the ferric ion.
Safety
Although unusual, consumption of oxalates (for example, the grazing of animals on oxalate-containing plants such as Bassia hyssopifolia, or human consumption of wood sorrel or, specifically in excessive quantities, black tea) may result in kidney disease or even death due to oxalate poisoning. The New England Journal of Medicine reported acute oxalate nephropathy "almost certainly due to excessive consumption of iced tea" in a 56-year-old man, who drank "sixteen 8-ounce glasses of iced tea daily". The authors of the paper hypothesized that acute oxalate nephropathy is an underdiagnosed cause of kidney failure and suggested thorough examination patient dietary history in cases of unexplained kidney failure without proteinuria (an excess of protein in the urine) and with large amounts of calcium oxalate in urine sediment.[21] Oxalobacter formigenes in the gut flora may help alleviate this.[22]
See also
- Dimethyl oxalate – (CH3)2C2O4
- Diphenyl oxalate – (C6H5)2C2O4
- Potassium ferrioxalate – K3[Fe(C2O4)3], an iron complex with oxalate ligands
- Raphide
- Sodium oxalate – Na2C2O4
References
- ↑ Riemenschneider, Wilhelm; Tanifuji, Minoru (2000). "Oxalic Acid". Ullmann's Encyclopedia of Industrial Chemistry. doi:10.1002/14356007.a18_247. ISBN 3-527-30673-0.
- 1 2 Dean, Philip A. W. (2012). "The Oxalate Dianion, C
2O2−
4: Planar or Nonplanar?". Journal of Chemical Education. 89 (3): 417–418. Bibcode:2012JChEd..89..417D. doi:10.1021/ed200202r. - ↑ Reed, D. A.; Olmstead, M. M. (1981). "Sodium oxalate structure refinement". Acta Crystallographica Section B. 37 (4): 938–939. doi:10.1107/S0567740881004676.
- ↑ Beagley, B.; Small, R. W. H. (1964). "The structure of lithium oxalate". Acta Crystallographica. 17 (6): 783–788. doi:10.1107/S0365110X64002079.
- ↑ In the figure 81(1)°, the (1) indicates that 1° is the standard uncertainty of the measured angle of 81°
- ↑ Dinnebier, Robert E.; Vensky, Sascha; Panthöfer, Martin; Jansen, Martin (2003). "Crystal and Molecular Structures of Alkali Oxalates: First Proof of a Staggered Oxalate Anion in the Solid State". Inorganic Chemistry. 42 (5): 1499–507. doi:10.1021/ic0205536. PMID 12611516.
- ↑ Clark, Timothy; Schleyer, Paul von Ragué (1981). "Conformational preferences of 34 valence electron A2X4 molecules: Anab initio Study of B2F4, B2Cl4, N2O4, and C
2O2−
4". Journal of Computational Chemistry. 2: 20–29. doi:10.1002/jcc.540020106. - ↑ Dewar, Michael J.S.; Zheng, Ya-Jun (1990). "Structure of the oxalate ion". Journal of Molecular Structure: THEOCHEM. 209: 157–162. doi:10.1016/0166-1280(90)85053-P.
- ↑ Herbert, John M.; Ortiz, J. V. (2000). "Ab Initio Investigation of Electron Detachment in Dicarboxylate Dianions". The Journal of Physical Chemistry A. 104 (50): 11786–11795. doi:10.1021/jp002657c.
- ↑ Streitweiser, Andrew, Jr.; Heathcock, Clayton H. (1976). Introduction to Organic Chemistry. Macmillan. p. 737.
- ↑ Resnick, Martin I.; Pak, Charles Y. C. (1990). Urolithiasis, A Medical and Surgical Reference. W.B. Saunders Company. p. 158. ISBN 0-7216-2439-1.
- ↑ Coe; Evan; Worcester (2005). "Kidney stone disease". The Journal of Clinical Investigation. 115 (10): 2598–608. doi:10.1172/JCI26662. PMC 1236703
. PMID 16200192.
- ↑ Betsche, T.; Fretzdorff, B. (2005). "Biodegradation of oxalic acid from spinach using cereal radicles". Journal of Agricultural and Food Chemistry. 53 (25): 9751–8. doi:10.1021/jf051091s. PMID 16332126.
- ↑ "UPMC Article, Low Oxalate Diet".
- ↑ "UMMC Condition Guide: Gout".
- ↑ Morozumi, Makoto; Hossain, Rayhan Zubair; Yamakawa, Ken'ichi; Hokama, Sanehiro; Nishijima, Saori; Oshiro, Yoshinori; Uchida, Atsushi; Sugaya, Kimio; Ogawa, Yoshihide (2006). "Gastrointestinal oxalic acid absorption in calcium-treated rats". Urological research. 34 (3): 168–72. doi:10.1007/s00240-006-0035-7. PMID 16705467.
- ↑ Hossain, R. Z.; Ogawa, Y.; Morozumi, M.; Hokama, S.; Sugaya, K. (2003). "Milk and calcium prevent gastrointestinal absorption and urinary excretion of oxalate in rats". Frontiers in Bioscience. 8 (1–3): a117–25. doi:10.2741/1083. PMID 12700095.
- ↑ Mandl; Szarka; Bánhegyi (2009). "Vitamin C: update on physiology and pharmacology". British Journal of Pharmacology. 157 (7): 1097–1110. doi:10.1111/j.1476-5381.2009.00282.x. PMC 2743829
. PMID 19508394.
- ↑ Pabuççuoğlu, Uğur (2005). "Aspects of oxalosis associated with aspergillosis in pathology specimens". Pathology – Research and Practice. 201 (5): 363–8. doi:10.1016/j.prp.2005.03.005. PMID 16047945.
- ↑ Lieske, J. C.; Goldfarb, D. S.; De Simone, C.; Regnier, C. (2005). "Use of a probiotic to decrease enteric hyperoxaluria". Kidney International. 68 (3): 1244–9. doi:10.1111/j.1523-1755.2005.00520.x. PMID 16105057.
- ↑ Syed, Fahd; Mena Gutiérrez, Alejandra; Ghaffar, Umbar (2 April 2015). "A Case of Iced-Tea Nephropathy". New England Journal of Medicine. 372 (14): 1377–1378. doi:10.1056/NEJMc1414481. PMID 25830441. Lay summary.
- ↑ Siener, R.; Bangen, U.; Sidhu, H.; Hönow, R.; von Unruh, G.; Hesse, A. (2013). "The role of Oxalobacter formigenes colonization in calcium oxalate stone disease". Kidney International. 83 (June): 1144–9. doi:10.1038/ki.2013.104. PMID 23536130.