Peak uranium
Peak uranium is the point in time that the maximum global uranium production rate is reached. After that peak, according to Hubbert peak theory, the rate of production enters a terminal decline. While uranium is used in nuclear weapons, its primary use is for energy generation via nuclear fission of the uranium-235 isotope in a nuclear power reactor.[1] Each kilogram of uranium-235 fissioned releases the energy equivalent of millions of times its mass in chemical reactants, as much energy as 2700 tons of coal, but uranium-235 is only 0.7% of the mass of natural uranium.[2] Uranium-235 is a finite non-renewable resource.[1][3] However, the current reserves of uranium have the potential (assuming breeder reactor technology) to provide power for humanity for billions of years, until the death of our sun, so nuclear power can be considered sustainable energy.[4]
M. King Hubbert created his peak theory in 1956 for a variety of finite resources such as coal, oil, and natural gas.[5] He and others since have argued that if the nuclear fuel cycle can be closed, uranium could become equivalent to renewable energy sources as concerns its availability.[6] Breeding and nuclear reprocessing potentially would allow the extraction of the largest amount of energy from natural uranium. However, only a small amount of uranium is currently being bred into plutonium and only a small amount of fissile uranium and plutonium is being recovered from nuclear waste worldwide. Furthermore, the technologies to completely eliminate the waste in the nuclear fuel cycle do not yet exist.[7] Since the nuclear fuel cycle is effectively not closed, Hubbert peak theory may be applicable.
Pessimistic predictions of future high-grade uranium production operate on the thesis that either the peak has already occurred in the 1980s or that a second peak may occur sometime around 2035.
At the start of 2013, identified uranium reserves recoverable at US$130/kg were 5.9 million tons.[8] At the rate of consumption in 2012, these reserves are sufficient for 120 years of supply.[8] The identified reserves as of 2013 recoverable at US$260/kg are 7.64 million tons.[8]
Optimistic predictions are based upon 3 factors:
- Light Water Reactors only consume about half of one percent of their uranium fuel while fast breeder reactors will consume closer to 99%,
- current reserves of U are about 5.3 million tons. Theoretically 4.5 billion tons of uranium are available from sea water at about 10 times the current price of uranium.[9] Currently no practical methods for high volume extraction exist.
- thorium (3-4 times as abundant as uranium) can be used when supplies of uranium are depleted.
If these predictions became reality it has the potential to increase the supply of nuclear fuel significantly.
Optimistic predictions claim that the supply is far more than demand and do not predict peak uranium.
Hubbert's peak and uranium
Uranium-235, the fissile isotope of uranium used in nuclear reactors, makes up about 0.72% of uranium from ore. It is the only naturally-occurring isotope capable of directly generating nuclear power, and is a finite, non-renewable resource. It is believed that its availability follows M. King Hubbert's peak theory, which was developed to describe peak oil. Hubbert saw oil as a resource which would soon run out, but he believed that uranium had much more promise as an energy source,[5] and that breeder reactors and nuclear reprocessing, which were new technologies at the time, would allow uranium to be a power source for a very long time. The technologies Hubbert envisioned would substantially reduce the rate of depletion of uranium-235, but they are still more costly than the "once-through" cycle, and have not been widely deployed to date.[10] If these and other more costly technologies such as seawater extraction are used, any possible peak would occur in the very distant future.
According to the Hubbert Peak Theory, Hubbert's peaks are the points where production of a resource, has reached its maximum, and from then on, the rate of resource production enters a terminal decline. After a Hubbert's peak, the rate of supply of a resource no longer fulfills the previous demand rate.[11] As a result of the law of supply and demand, at this point the market shifts from a buyer's market[12] to a seller's market.[13]
Many countries are not able to supply their own uranium demands any longer and must import uranium from other countries. Thirteen countries have hit peak and exhausted their uranium resources.[14][15]
Uranium demand
The world demand for uranium in 1996 was over 68 kilotonnes (150×10 6 lb) per year,[17] and that number had been expected to increase to between 80 kilotonnes (180×10 6 lb) and 100 kilotonnes (220×10 6 lb) per year by 2025 due to the number of new nuclear power plants coming on line.[18] However following the shutdown of many nuclear power plants after the Fukushima Daiichi nuclear disaster in 2011, demand had fallen to about 60 kilotonnes (130×10 6 lb) per year in 2015 with future forecasts uncertain.[19]
According to Cameco Corporation, the demand for uranium is directly linked to the amount of electricity generated by nuclear power plants. Reactor capacity is growing slowly, reactors are being run more productively, with higher capacity factors, and reactor power levels. Improved reactor performance translates into greater uranium consumption.[20]
Nuclear power stations of 1000 megawatt electrical generation capacity require around 200 tonnes (440×10 3 lb) of uranium per year. For example, the United States has 103 operating reactors with an average generation capacity of 950 MWe demanded over 22 kilotonnes (49×10 6 lb) of uranium in 2005.[21] As the number of nuclear power plants increase, so does the demand for uranium.
Another factor to consider is population growth. Electricity consumption is determined in part by economic and population growth. According to data from the CIA's World Factbook, the world population currently (July 2012 est.) is more than 7 billion and it is increasing by 1.167% per year. This means a growth of about 211,000 persons every day.[22] According to the UN, by 2050 it is estimated that the Earth's population will be 9.07 billion.[23] That's 37% increase from today. 62% of the people will live in Africa, Southern Asia and Eastern Asia.[24] The largest energy-consuming class in the history of earth is being produced in world’s most populated countries, China and India. Both plan massive nuclear energy expansion programs. China intends to build 32 nuclear plants with 40,000 MWe capacity by 2020.[25] According to the World Nuclear Association, India plans on bringing 20,000 MWe nuclear capacity on line by 2020, and aims to supply 25% of electricity from nuclear power by 2050.[26] The World Nuclear Association believes nuclear energy could reduce the fossil fuel burden of generating the new demand for electricity.[27]
As more fossil fuels are used to supply the growing energy needs of an increasing population, the more greenhouse gases are produced. Some proponents of nuclear power believe that building more nuclear power plants can reduce greenhouse emissions.[28] For example, the Swedish utility Vattenfall studied the full life cycle emissions of different ways to produce electricity, and concluded that nuclear power produced 3.3 g/kWh of carbon dioxide, compared to 400.0 for natural gas and 700.0 for coal.[29] However, more recent studies have shown that coal produces closer to 1000 g/kWh of carbon dioxide, and that nuclear powers emissions are comparable to conventional renewable energy sources, with both being in the range of ~16 g/kWh.
As countries are not able to supply their own needs economically from their own mines have resorted to importing better grades of uranium from elsewhere. For example, owners of U.S. nuclear power reactors bought 67 million pounds (30 kt) of uranium in 2006. Out of that 84%, or 56 million pounds (25 kt), were imported from foreign suppliers, according to the Energy Department.[30]
Because of the improvements in gas centrifuge technology in the 2000s, replacing former gaseous diffusion plants, cheaper separative work units have enabled the economic production of more enriched uranium from a given amount of natural uranium, by re-enriching tails ultimately leaving a depleted uranium tail of lower enrichment. This has somewhat lowered the demand for natural uranium.[19]
Uranium supply
Uranium occurs naturally in many rocks, and even in seawater. However, like other metals, it is seldom sufficiently concentrated to be economically recoverable.[31] Like any resource, uranium cannot be mined at any desired concentration. No matter the technology, at some point it is too costly to mine lower grade ores. One highly criticized[32] life cycle study by Jan Willem Storm van Leeuwen suggested that below 0.01–0.02% (100–200 ppm) in ore, the energy required to extract and process the ore to supply the fuel, operate reactors and dispose properly comes close to the energy gained by burning the uranium in the reactor.[33] Researchers at the Paul Scherrer Institute who analyzed the Jan Willem Storm van Leeuwen paper however have detailed the number of incorrect assumptions of Jan Willem Storm van Leeuwen that led them to this evaluation, including their assumption that all the energy used in the mining of Olympic Dam is energy used in the mining of uranium, when that mine is predominantly a copper mine and uranium is produced only as a co-product, along with gold and other metals.[32] The report by Jan Willem Storm van Leeuwen also assumes that all enrichment is done in the older and more energy intensive gaseous diffusion technology, however the less energy intensive gas centrifuge technology has produced the majority of the world's enriched uranium now for a number of decades.
An appraisal of nuclear power by a team at MIT in 2003, and updated in 2009, have stated that:[34]
Most commentators conclude that a half century of unimpeded growth is possible, especially since resources costing several hundred dollars per kilogram (not estimated in the Red Book) would also be economically usable...We believe that the world-wide supply of uranium ore is sufficient to fuel the deployment of 1000 reactors over the next half century.
In the early days of the nuclear industry, uranium was thought to be very scarce, so a closed fuel cycle would be needed. Fast breeder reactors would be needed to create nuclear fuel for other power producing reactors. In the 1960s, new discoveries of reserves, and new uranium enrichment techniques allayed these concerns.[35]
Mining companies usually consider concentrations greater than 0.075% (750 ppm) as ore, or rock economical to mine at current uranium market prices.[36] There is around 40 trillion tons of uranium in Earth's crust, but most is distributed at low parts per million trace concentration over its 3 * 1019 ton mass.[37][38] Estimates of the amount concentrated into ores affordable to extract for under $130 per kg can be less than a millionth of that total.[14]
Source | Concentration |
---|---|
Very high-grade ore – 20% U | 200,000 ppm U |
High-grade ore – 2% U | 20,000 ppm U |
Low-grade ore – 0.1% U | 1,000 ppm U |
Very low-grade ore – 0.01% U | 100 ppm U |
Granite | 4–5 ppm U |
Sedimentary rock | 2 ppm U |
Earth's continental crust (av) | 2.8 ppm U |
Seawater | 0.003 ppm U |
According to the OECD Redbook, the world consumed 67 kilotonnes (150×10 6 lb) of uranium in 2002. Of that, 36 kilotonnes (79×10 6 lb) was produced from primary sources, with the balance coming from secondary sources, in particular stockpiles of natural and enriched uranium, decommissioned nuclear weapons, the reprocessing of natural and enriched uranium and the re-enrichment of depleted uranium tails.[40]
Ore concentration | tonnes of uranium | Ore type |
---|---|---|
> 1% | 10000 | vein deposits |
0.2% – 1% | 2 million | pegmatites,unconformity deposits |
0.1% – 0.2% | 80 million | fossil placers, sandstones |
0.02% – 0.1% | 100 million | lower grade fossil placers, sandstones |
100–200 ppm | 2 billion | volcanic deposits |
The table above assumes the fuel will be used in a LWR burner. Uranium becomes far more economical when used in a fast burner reactor such as the Integral Fast Reactor.
Production


Peak uranium refers to the peak of the entire planet's uranium production. Like other Hubbert peaks, the rate of uranium production on Earth will enter a terminal decline. According to Robert Vance of the OECD's Nuclear Energy Agency, the world production rate of uranium has already reached its peak in 1980, amounting to 69,683 tonnes (150×10 6 lb) of U3O8 from 22 countries. However, this is not due to lack of production capacity. Historically, uranium mines and mills around the world have operated at about 76% of total production capacity, varying within a range of 57% and 89%. The low production rates have been largely attributable to excess capacity. Slower growth of nuclear power and competition from secondary supply significantly reduced demand for freshly mined uranium until very recently. Secondary supplies include military and commercial inventories, enriched uranium tails, reprocessed uranium and mixed oxide fuel.[43]
According to data from the International Atomic Energy Agency, world production of mined uranium has peaked twice in the past: once, circa 1960 in response to stockpiling for military use, and again in 1980, in response to stockpiling for use in commercial nuclear power. Up until about 1990, the mined uranium production was in excess of consumption by power plants. But since 1990, consumption by power plants has outstripped the uranium being mined; the deficit being made up by liquidation of the military (through decommissioning of nuclear weapons) and civilian stockpiles. Uranium mining has increased since the mid-1990s, but is still less than the consumption by power plants.[44]
The world's top uranium producers are Canada (28% of world production) and Australia (23%). Other major producers include Kazakhstan, Russia, Namibia and Niger.[45] In 1996, the world produced 39 kilotonnes (86×10 6 lb) of uranium.[46] In 2005, the world produced a peak of 41,720 tonnes (92×10 6 lb) of uranium,[42] although the production continues not to meet demand. Only 62% of the requirements of power utilities are supplied by mines. The balance comes from inventories held by utilities and other fuel cycle companies, inventories held by governments, used reactor fuel that has been reprocessed, recycled materials from military nuclear programs and uranium in depleted uranium stockpiles.[47] The plutonium from dismantled Cold War nuclear weapon stockpiles will be exhausted by 2013. The industry is trying to find and develop new uranium mines, mainly in Canada, Australia and Kazakhstan. Those under development in 2006 would fill half the gap.[48]
Of the ten largest uranium mines in the world (Mc Arthur River, Ranger, Rossing, Kraznokamensk, Olympic Dam, Rabbit Lake, Akouta, Arlit, Beverly, and McClean Lake), by 2020, six will be depleted, two will be in their final stages, one will be upgrading and one will be producing.[49]
World primary mining production fell 5% in 2006 over that in 2005. The biggest producers, Canada and Australia saw falls of 15% and 20%, with only Kazakhstan showing an increase of 21%.[50] This can be explained by two major events that have slowed world uranium production. Canada's Cameco mine at Cigar Lake is the largest, highest-grade uranium mine in the world. In 2006 it flooded, and then flooded again in 2008 (after Cameco had spent $43 million—most of the money set aside—to correct the problem), causing Cameco to push back its earliest start-up date for Cigar Lake to 2011.[51] Also, in March 2007, the market endured another blow when a cyclone struck the Ranger mine in Australia, which produces 5,500 tonnes (12×10 6 lb) of uranium a year. The mine's owner, Energy Resources of Australia, declared force majeure on deliveries and said production would be impacted into the second half of 2007.[52] This caused some to speculate that peak uranium has arrived.[53]
Primary sources
About 96% of the global uranium reserves are found in these ten countries: Australia, Canada, Kazakhstan, South Africa, Brazil, Namibia, Uzbekistan, the United States, Niger, and Russia[54] Out of those Canada (28% of world production) and Australia (23%) are the major producers.[45] In 1996, the world produced 39,000 tonnes of uranium,[55] and in 2005, the world produced a peak of 41,720 tonnes of uranium,[42] although the production continues to not meet demand.
Various agencies have tried to estimate how long these primary resources will last, assuming a once-through cycle. The European Commission said in 2001 that at the current level of uranium consumption, known uranium resources would last 42 years. When added to military and secondary sources, the resources could be stretched to 72 years. Yet this rate of usage assumes that nuclear power continues to provide only a fraction of the world’s energy supply. If electric capacity were increased six-fold, then the 72-year supply would last just 12 years.[56] The world's present measured resources of uranium, economically recoverable at a price of US$130/kg according to the industry groups Organisation for Economic Co-operation and Development (OECD), Nuclear Energy Agency (NEA) and International Atomic Energy Agency (IAEA), are enough to last for "at least a century" at current consumption rates.[57][8] According to the World Nuclear Association, yet another industry group, assuming the world's current rate of consumption at 66,500 tonnes of uranium per year and the world's present measured resources of uranium (4.7 Mt – 5.5 Mt[57]) are enough to last for some 70 – 80 years.[58]
Reserves
Reserves are the most readily available resources. Resources that are known to exist and easy to mine are called "Known conventional resources". Resources that are thought to exist but have not been mined are classified under "Undiscovered conventional resources".[59]
The known uranium resources represent a higher level of assured resources than is normal for most minerals. Further exploration and higher prices will certainly, on the basis of present geological knowledge, yield further resources as present ones are used up. There was very little uranium exploration between 1985 and 2005, so the significant increase in exploration effort that we are now seeing could readily double the known economic resources. On the basis of analogies with other metal minerals, a doubling of price from price levels in 2007 could be expected to create about a tenfold increase in measured resources, over time.[60]
Known conventional resources
Known conventional resources are "Reasonably Assured Resources" and "Estimated Additional Resources-I".[59]
In 2006, about 4 million tons of conventional resources were thought to be sufficient at current consumption rates for about six decades (4.06 million tonnes at 65,000 tones per year).[61] In 2011, this was estimated to be 7 million tonnes. Exploration for uranium has increased. From 1981 to 2007, annual exploration expenditures grew modestly, from 4 million US$ to 7 million US$. This skyrocketed to US $11 million in 2011.[35] Consumption of uranium runs at around 75 000 t a year. This is less than production, and requires draw down of existing stocks.
About 96% of the global uranium reserves are found in these ten countries: Australia, Canada, Kazakhstan, South Africa, Brazil, Namibia, Uzbekistan, the United States, Niger, and Russia.[54] The world's largest deposits of uranium are found in three countries. Australia has just over 30% of the world's reasonably assured resources and inferred resources of uranium – about 1.673 megatonnes (3.69×10 9 lb).[31] Kazakhstan has about 12% of the world's reserves, or about 651 kilotonnes (1.4×10 9 lb).[58] And Canada has 485 kilotonnes (1,100×10 6 lb) of uranium, representing about 9%.[31]
Several countries in Europe no longer mine uranium (East Germany (1990), France (2001), Spain (2002) and Sweden (1969)); they were not major producers.[15]
Undiscovered conventional resources
Undiscovered conventional resources can be broken up into two classifications "Estimated Additional Resources-II" and "Speculative Resources".[59]
It will take a significant exploration and development effort to locate the remaining deposits and begin mining them. However, since the entire earth's geography has not been explored for uranium at this time, there is still the potential to discover exploitable resources.[62] The OECD Redbook cites areas still open to exploration throughout the world. Many countries are conducting complete aeromagnetic gradiometer radiometric surveys to get an estimate the size of their undiscovered mineral resources. Combined with a gamma-ray survey, these methods can locate undiscovered uranium and thorium deposits.[63] The U.S. Department of Energy conducted the first and only national uranium assessment in 1980 – the National Uranium Resource Evaluation (NURE) program.[64]
Secondary resources
Secondary resources are essentially recovered uranium from other sources such as nuclear weapons, inventories, reprocessing and re-enrichment. Since secondary resources have exceedingly low discovery costs and very low production costs, they may have displaced a significant portion of primary production. Secondary uranium was and is available essentially instantly. However, new primary production will not be. Essentially, secondary supply is a "one-time" finite supply.[65]
Only 62% of the requirements of power utilities are supplied by mines. The balance comes from inventories held by utilities and other fuel cycle companies, inventories held by governments, used reactor fuel that has been reprocessed, recycled materials from military nuclear programs and uranium in depleted uranium stockpiles.[66]
The plutonium from dismantled cold war nuclear weapon stockpiles dried up in 2013. The industry is trying to find and develop new uranium mines, mainly in Canada, Australia and Kazakhstan. However, those under development will fill only half the current gap.[48]
Inventories
Inventories are kept by a variety of organizations - government, commercial and others.[67][68]
The US DOE keeps inventories for security of supply in order to cover for emergencies where uranium is not available at any price.[69] In the event of a major supply disruption, the Department may not have sufficient uranium to meet a severe uranium shortage in the United States.
Decommissioning nuclear weapons
Both the US and Russia have committed to recycle their nuclear weapons into fuel for electricity production. This program is known as the Megatons to Megawatts Program. [70] Down blending 500 tonnes (1,100×10 3 lb) of Russian weapons high enriched uranium (HEU) will result in about 15 kilotonnes (33,000×10 3 lb) of low enriched uranium (LEU) over 20 years. This is equivalent to about 152 kilotonnes (340×10 6 lb) of natural U, or just over twice annual world demand. Since 2000, 30 tonnes (66×10 3 lb) of military HEU is displacing about 10.6 kilotonnes (23×10 6 lb) of uranium oxide mine production per year which represents some 13% of world reactor requirements.[71]
Plutonium recovered from nuclear weapons or other sources can be blended with uranium fuel to produce a mixed-oxide fuel. In June 2000, the USA and Russia agreed to dispose of 34 kilotonnes (75×10 6 lb) each of weapons-grade plutonium by 2014. The US undertook to pursue a self-funded dual track program (immobilization and MOX). The G-7 nations provided US$1 billion to set up Russia's program. The latter was initially MOX specifically designed for VVER reactors, the Russian version of the Pressurized Water Reactor (PWR), the high cost being because this was not part of Russia's fuel cycle policy. This MOX fuel for both countries is equivalent to about 12 kilotonnes (26×10 6 lb) of natural uranium.[72] The U.S. also has commitments to dispose of 151 tonnes (330×10 3 lb) of non-waste HEU.[73]
The Megatons to Megawatts program came to an end in 2013.[70]
Reprocessing and recycling
Nuclear reprocessing, sometimes called recycling, is one method of mitigating the eventual peak of uranium production. It is most useful as part of a nuclear fuel cycle utilizing fast-neutron reactors since reprocessed uranium and reactor-grade plutonium both have isotopic compositions not optimal for use in today's thermal-neutron reactors. Although reprocessing of nuclear fuel is done in a few countries (France, United Kingdom, and Japan) the United States President banned reprocessing in the late 1970s due to the high costs and the risk of nuclear proliferation via plutonium. In 2005, U.S. legislators proposed a program to reprocess the spent fuel that has accumulated at power plants. At present prices, such a program is significantly more expensive than disposing spent fuel and mining fresh uranium.[10]
Currently, there are eleven reprocessing plants in the world. Of these, two are large-scale commercially operated plants for the reprocessing of spent fuel elements from light water reactors with throughputs of more than 1 kilotonne (2.2×10 6 lb) of uranium per year. These are La Hague, France with a capacity of 1.6 kilotonnes (3.5×10 6 lb) per year and Sellafield, England at 1.2 kilotonnes (2.6×10 6 lb) uranium per year. The rest are small experimental plants.[74] The two large-scale commercial reprocessing plants together can reprocess 2,800 tonnes of uranium waste annually.[75]
Most of the spent fuel components can be recovered and recycled. About two-thirds of the U.S. spent fuel inventory is uranium. This includes residual fissile uranium-235 that can be recycled directly as fuel for heavy water reactors or enriched again for use as fuel in light water reactors.[76]
Plutonium and uranium can be chemically separated from spent fuel. When used nuclear fuel is reprocessed using the de facto standard PUREX method, both plutonium and uranium are recovered separately. The spent fuel contains about 1% plutonium. Reactor-grade plutonium contains Pu-240 which has a high rate of spontaneous fission, making it an undesirable contaminant in producing safe nuclear weapons. Nevertheless, nuclear weapons can be made with reactor grade plutonium.[77]
The spent fuel is primarily composed of uranium, most of which has not been consumed or transmuted in the nuclear reactor. At a typical concentration of around 96% by mass in the used nuclear fuel, uranium is the largest component of used nuclear fuel.[78] The composition of reprocessed uranium depends on the time the fuel has been in the reactor, but it is mostly uranium-238, with about 1% uranium-235, 1% uranium-236 and smaller amounts of other isotopes including uranium-232. However, reprocessed uranium is also a waste product because it is contaminated and undesirable for reuse in reactors.[79] During its irradiation in a reactor, uranium is profoundly modified. The uranium that leaves the reprocessing plant contains all the isotopes of uranium between uranium-232 and uranium-238 except uranium-237, which is rapidly transformed into neptunium-237. The undesirable isotopic contaminants are:
- Uranium-232 (whose decay products emit strong gamma radiation making handling more difficult), and
- Uranium-234 (which is fertile material but can affect reactivity differently from uranium-238).
- Uranium-236 (which affects reactivity and absorbs neutrons without fissioning, becoming neptunium-237 which is one of the most difficult isotopes for long-term disposal in a deep geological repository)
- Daughter products of uranium-232: bismuth-212, thallium-208.[80]
At present, reprocessing and the use of plutonium as reactor fuel is far more expensive than using uranium fuel and disposing of the spent fuel directly—even if the fuel is only reprocessed once.[79] However, nuclear reprocessing becomes more economically attractive, compared to mining more uranium, as uranium prices increase.
The total recovery rate 5 kilotonnes (11×10 6 lb)/yr from reprocessing currently is only a small fraction compared to the growing gap between the rate demanded 64.615 kilotonnes (142.45×10 6 lb)/yr and the rate at which the primary uranium supply is providing uranium 46.403 kilotonnes (102.30×10 6 lb)/yr.
Energy Returned on Energy Invested (EROEI) on uranium reprocessing is highly positive, though not as positive as the mining and enrichment of uranium, and the process can be repeated. Additional reprocessing plants may bring some economies of scale.
The main problems with uranium reprocessing are the cost of mined uranium compared to the cost of reprocessing,[10][81] nuclear proliferation risks, the risk of major policy change, the risk of incurring large cleanup costs, stringent regulations for reprocessing plants, and the anti-nuclear movement .
Unconventional resources
Unconventional resources are occurrences that require novel technologies for their exploitation and/or use. Often unconventional resources occur in low-concentration. The exploitation of unconventional uranium requires additional research and development efforts for which there is no imminent economic need, given the large conventional resource base and the option of reprocessing spent fuel.[82] Phosphates, seawater, uraniferous coal ash, and some type of oil shales are examples of unconventional uranium resources.
Phosphates
The soaring price of uranium may cause long-dormant operations to extract uranium from phosphate. Uranium occurs at concentrations of 50 to 200 parts per million in phosphate-laden earth or phosphate rock. As uranium prices increase, there has been interest in some countries in extraction of uranium from phosphate rock, which is normally used as the basis of phosphate fertilizers.[83]
Worldwide, approximately 400 wet-process phosphoric acid plants were in operation. Assuming an average recoverable content of 100 ppm of uranium, and that uranium prices do not increase so that the main use of the phosphates are for fertilizers, this scenario would result in a maximum theoretical annual output of 3.7 kilotonnes (8.2×10 6 lb) U3O8.[84]
Historical operating costs for the uranium recovery from phosphoric acid range from $48–$119/kg U3O8.[85] In 2011, the average price paid for U3O8 in the United States was $122.66/kg.[86]
There are 22 million tons of uranium in phosphate deposits. Recovery of uranium from phosphates is a Mature technology;[82] it has been utilized in Belgium and the United States, but high recovery costs limit the utilization of these resources, with estimated production costs in the range of US$60–100/kgU including capital investment, according to a 2003 OECD report for a new 100 tU/year project.[40]
Seawater
Unconventional uranium resources include up to 4,000 megatonnes (8,800×10 9 lb) of uranium contained in sea water. The technology to extract uranium from sea water has only been demonstrated at the laboratory scale, and extraction costs were estimated in the mid-1990s at US$260/kgU (Nobukawa, et al., 1994) but scaling up laboratory-level production to thousands of tonnes is unproven and may encounter unforeseen difficulties.[87]
One method of extracting uranium from seawater is using a uranium-specific nonwoven fabric as an absorbent. The total amount of uranium recovered in an experiment in 2003 from three collection boxes containing 350 kg of fabric was >1 kg of yellow cake after 240 days of submersion in the ocean.[88] According to the OECD, uranium may be extracted from seawater using this method for about US$300/kgU [40]
In 2006 the same research group stated: "If 2g-U/kg-adsorbent is submerged for 60 days at a time and used 6 times, the uranium cost is calculated to be 88,000 yen/kg-U, including the cost of adsorbent production, uranium collection, and uranium purification. When 6g-U/kg-adsorbent and 20 repetitions or more becomes possible, the uranium cost reduces to 15,000 yen. This price level is equivalent to that of the highest cost of the minable uranium. The lowest cost attainable now is 25,000 yen with 4g-U/kg-adsorbent used in the sea area of Okinawa, with 18 repetition uses. In this case, the initial investment to collect the uranium from seawater is 107.7 billion yen, which is 1/3 of the construction cost of a one million-kilowatt class nuclear power plant."[89]
In 2012, ORNL researchers announced the successful development of a new absorbent material dubbed HiCap, which vastly outperforms previous best adsorbents, which perform surface retention of solid or gas molecules, atoms or ions. "We have shown that our adsorbents can extract five to seven times more uranium at uptake rates seven times faster than the world's best adsorbents," said Chris Janke, one of the inventors and a member of ORNL's Materials Science and Technology Division. HiCap also effectively removes toxic metals from water, according to results verified by researchers at Pacific Northwest National Laboratory.[90][91][92][93][94]
Among the other methods to recover uranium from sea water, two seem promising: algae bloom to concentrate uranium[95] and nanomembrane filtering.[96]
So far, no more than a very small amount of uranium has been recovered from sea water in a laboratory.[82]
Uraniferous coal ash

In particular, nuclear power facilities produce about 200,000 metric tons of low and intermediate level waste (LILW) and 10,000 metric tons of high level waste (HLW) (including spent fuel designated as waste) each year worldwide.[99]
Although only several parts per million average concentration in coal before combustion (albeit more concentrated in ash), the theoretical maximum energy potential of trace uranium and thorium in coal (in breeder reactors) actually exceeds the energy released by burning the coal itself, according to a study by Oak Ridge National Laboratory.[98]
From 1965 to 1967 Union Carbide operated a mill in North Dakota, United States burning uraniferous lignite and extracting uranium from the ash. The plant produced about 150 metric tons of U3O8 before shutting down.[100]
An international consortium has set out to explore the commercial extraction of uranium from uraniferous coal ash from coal power stations located in Yunnan province, China.[82] The first laboratory scale amount of yellowcake uranium recovered from uraniferous coal ash was announced in 2007.[101] The three coal power stations at Xiaolongtang, Dalongtang and Kaiyuan have piled up their waste ash. Initial tests from the Xiaolongtang ash pile indicate that the material contains (160–180 parts per million uranium), suggesting a total of some 2.085 kilotonnes (4.60×10 6 lb) U3O8 could be recovered from that ash pile alone.[101]
Oil shales
Some oil shales contain uranium, which may be recovered as a byproduct. Between 1946 and 1952, a marine type of Dictyonema shale was used for uranium production in Sillamäe, Estonia, and between 1950 and 1989 alum shale was used in Sweden for the same purpose.[102]
Breeding
A breeder reactor produces more nuclear fuel than it consumes and thus can extend the uranium supply. It typically turns the dominant isotope in natural uranium, uranium-238, into fissile plutonium-239. This results in hundredfold increase in the amount of energy to be produced per mass unit of uranium, because U-238, which constitute 99.3% of natural uranium, is not used in conventional reactors which instead use U-235 which only represent 0.7% of natural uranium.[103] In 1983, physicist Bernard Cohen proposed that the world supply of uranium is effectively inexhaustible, and could therefore be considered a form of renewable energy.[6][104] He claims that fast breeder reactors, fueled by naturally-replenished uranium-238 extracted from seawater, could supply energy at least as long as the sun's expected remaining lifespan of five billion years.,[6] making them as sustainable in fuel availability terms as renewable energy sources.
There are two types of breeders: Fast breeders and thermal breeders.
Fast breeder
A fast breeder, in addition to consuming U-235, converts fertile U-238 into Pu-239, a fissile fuel. Fast breeder reactors are more expensive to build and operate, including the reprocessing, and could only be justified economically if uranium prices were to rise to pre-1980 values in real terms. About 20 fast-neutron reactors have already been operating, some since the 1950s, and one supplies electricity commercially. Over 300 reactor-years of operating experience have been accumulated. In addition to considerably extending the exploitable fuel supply, these reactors have an advantage in that they produce less long-lived transuranic wastes, and can consume nuclear waste from current light water reactors, generating energy in the process.[105] Several countries have research and development programs for improving these reactors. For instance, one scenario in France is for half of the present nuclear capacity to be replaced by fast breeder reactors by 2050. China, India, and Japan plan large scale utilization of breeder reactors during the coming decades.[106] (Following the crisis at Japan's Fukishima Daiichi nuclear power plant in 2011, Japan is revising its plans regarding future use of nuclear power. (See: Fukushima Daiichi nuclear disaster: Energy policy implications.))
The breeding of plutonium fuel in Fast Breeder Reactors (FBR), known as the plutonium economy, was for a time believed to be the future of nuclear power. But many of the commercial breeder reactors that have been built have been riddled with technical and budgetary problems. Some sources critical of breeder reactors have gone so far to call them the Supersonic Transport of the '80s.[107]
Uranium turned out to be far more plentiful than anticipated, and the price of uranium declined rapidly (with an upward blip in the 1970s). This is why the US halted their use in 1977[108] and the UK abandoned the idea in 1994.[109]
Fast Breeder Reactors, are called fast because they have no moderator slowing down the neutrons (light water, heavy water or graphite) and breed more fuel than they consume. The word 'fast' in fast breeder thus refers to the speed of the neutrons in the reactor's core. The higher the energy the neutrons have, the higher the breeding ratio or the more uranium that is changed into plutonium.
Significant technical and materials problems were encountered with FBRs, and geological exploration showed that scarcity of uranium was not going to be a concern for some time. By the 1980s, due to both factors, it was clear that FBRs would not be commercially competitive with existing light water reactors. The economics of FBRs still depend on the value of the plutonium fuel which is bred, relative to the cost of fresh uranium.[110] Research continues in several countries with working prototypes Phénix in France, the BN-600 reactor in Russia, and the Monju scheduled to be restarted in 2009.[111]
On February 16, 2006 the United States, France and Japan signed an arrangement to research and develop sodium-cooled fast breeder reactors in support of the Global Nuclear Energy Partnership.[112] Breeder reactors are also being studied under the Generation IV reactor program.
Early prototypes have been plagued with problems. The liquid sodium coolant is highly flammable, bursting into flames if it comes into contact with air and exploding if it comes into contact with water. Japan's fast breeder Monju Nuclear Power Plant has been scheduled to re-open in 2008, 13 years after a serious accident and fire involving a sodium leak. In 1997 France shut down its Superphenix reactor, while the Phenix, built earlier, closed as scheduled in 2009.[113] [114]
At higher uranium prices breeder reactors may be economically justified. Many nations have ongoing breeder research programs. China, India, and Japan plan large scale utilization of breeder reactors during the coming decades. 300 reactor-years experience has been gained in operating them.[106]
As of June 2008 there are only two running commercial breeders and the rate of reactor-grade plutonium production is very small (20 tonnes/yr). The reactor grade plutonium is being processed into MOX fuel. However, next to the rate at which uranium is being mined (46,403 tonnes/yr), this is not enough to stave off Peak uranium.
Thermal breeder
Thorium is an alternate fuel cycle to uranium. Thorium is three times more plentiful than uranium. Thorium-232 is in itself not fissile, but fertile. It can be made into fissile uranium-233 in a breeder reactor. In turn, the uranium-233 can be fissioned, with the advantage that smaller amounts of transuranics are produced by neutron capture, compared to uranium-235 and especially compared to plutonium-239.
Despite the thorium fuel cycle having a number of attractive features, development on a large scale can run into difficulties:[115]
- The resulting U-233 fuel is expensive to fabricate.
- The U-233 chemically separated from the irradiated thorium fuel is highly radioactive.
- Separated U-233 is always contaminated with traces of U-232
- Thorium is difficult to recycle due to highly radioactive Th-228
- If the U-233 can be separated on its own, it becomes a weapons proliferation risk
- And, there are technical problems in reprocessing.
Advocates for liquid core and molten salt reactors such as LFTR claim that these technologies negate the abovementioned thorium's disadvantages present in solid fueled reactors.
The first successful commercial reactor at the Indian Point power station in Buchanan, New York (Indian Point Unit 1) ran on Thorium. The first core did not live up to expectations.[116]
Indian interest in thorium is motivated by their substantial reserves. Almost a third of the world's thorium reserves are in India.[117] India's Department of Atomic Energy (DAE) says that it will construct a 500 MWe prototype reactor in Kalpakkam. There are plans for four breeder reactors of 500 MWe each - two in Kalpakkam and two more in a yet undecided location.[118]
China has initiated a research and development project in thorium molten-salt breeder reactor technology.[119] It was formally announced at the Chinese Academy of Sciences (CAS) annual conference in January 2011. Its ultimate target is to investigate and develop a thorium based molten salt breeder nuclear system in about 20 years.[120][121][122] A 5 MWe research MSR is apparently under construction at Shanghai Institute of Applied Physics (under the Academy) with 2015 target operation.[123]
Supply-demand gap
Due to reduction in nuclear weapons stockpiles, a large amount of former weapons uranium was released for use in civilian nuclear reactors. As a result, starting in 1990, a significant portion of uranium nuclear power requirements were supplied by former weapons uranium, rather than newly mined uranium. In 2002, mined uranium supplied only 54 percent of nuclear power requirements.[124] But as the supply of former weapons uranium has been used up, mining has increased, so that in 2012, mining provided 95 percent of reactor requirements, and the OCED Nuclear Energy Agency and the International Atomic Energy Agency projected that the gap in supply would be completely erased in 2013.[8][125]
Country | Uranium required 2006-08[126] | % of world demand | Indigenous mining production 2006[127] | Deficit (-surplus) |
---|---|---|---|---|
![]() | 18,918 tonnes (42×10 6 lb) | 29.3% | 2,000 tonnes (4.4×10 6 lb) | 16,918 tonnes (37×10 6 lb) |
![]() | 10,527 tonnes (23×10 6 lb) | 16.3% | 0 | 10,527 tonnes (23×10 6 lb) |
![]() | 7,659 tonnes (17×10 6 lb) | 11.8% | 0 | 7,659 tonnes (17×10 6 lb) |
![]() | 3,365 tonnes (7.4×10 6 lb) | 5.2% | 4,009 tonnes (8.8×10 6 lb) | −644 tonnes (−1.4×10 6 lb) |
![]() | 3,332 tonnes (7.3×10 6 lb) | 5.2% | 68.03 tonnes (0.1500×10 6 lb) | 3,264 tonnes (7.2×10 6 lb) |
![]() | 3,109 tonnes (6.9×10 6 lb) | 4.8% | 0 | 3,109 tonnes (6.9×10 6 lb) |
![]() | 2,199 tonnes (4.8×10 6 lb) | 3.4% | 0 | 2,199 tonnes (4.8×10 6 lb) |
Rest of the World | 15,506 tonnes (34×10 6 lb) | 24.0% | 40,327 tonnes (89×10 6 lb) | −24,821 tonnes (−55×10 6 lb) |
Total | 64,615 tonnes (140×10 6 lb) | 100.0% | 46,403 tonnes (100×10 6 lb) | 18,211 tonnes (40×10 6 lb) |
Peak uranium for individual nations
Eleven countries, Germany, the Czech Republic, France, DR Congo, Gabon, Bulgaria, Tajikistan, Hungary, Romania, Spain, Portugal and Argentina, have seen uranium production peak, and rely on imports for their nuclear programs.[14][15] Other countries have reached their peak production of uranium and are currently on a decline.
- Germany – Between 1946 and 1990, Wismut, the former East German uranium mining company, produced a total of around 220 kilotonnes (490×10 6 lb) of uranium. During its peak, production exceeded 7 kilotonnes (15×10 6 lb) per year. In 1990, uranium mining was discontinued as a consequence of the German unification.[14] The company could not compete on the world market. The production cost of its uranium was three times the world price.[128]
- India – having already hit its production peak, India is finding itself in making a tough choice between using its modest and dwindling uranium resources as a source to keep its weapons programs rolling or it can use them to produce electricity.[129] Since India has abundant thorium reserves, it is switching to nuclear reactors powered by the thorium fuel cycle.
- Sweden – Sweden started uranium production in 1965 but was never profitable. They stopped mining uranium in 1969.[130] Sweden then embarked on a massive project based on American light water reactors. Nowadays, Sweden imports its uranium mostly from Canada, Australia and the former Soviet Union.
- UK – 1981: The UK's uranium production peaked in 1981 and the supply is running out. Yet the UK still plans to build more nuclear power plants.[48]
- France – 1988: In France uranium production attained a peak of 3,394 tonnes (7.5×10 6 lb) in 1988. At the time, this was enough for France to meet the half of its reactor demand from domestic sources.[131] By 1997, production was 1/5 of the 1991 levels. France markedly reduced its market share since 1997.[132] In 2002, France ran out of uranium.[127]

- U.S. – 1980: The United States was the world's leading producer of uranium from 1953 until 1980, when annual US production peaked at 16,810 tonnes (37×10 6 lb) (U3O8) according to the OECD redbook.[133] According to the CRB yearbook, US production the peak was at 19,822 tonnes (44×10 6 lb).[134] The U.S. production hit another maximum in 1996 at 6.3 million pounds (2.9 kt) of uranium oxide (U3O8), then dipped in production for a few years.[135] Between 2003 and 2007, there has been a 125% increase in production as demand for uranium has increased. However, as of 2008, production levels have not come back to 1980 levels.
Year | 1993 | 1994 | 1995 | 1996 | 1997 | 1998 | 1999 | 2000 | 2001 | 2002 | 2003 | 2004 | 2005 | 2006 | 2007 | 2008 | 2009 |
U3O8 (Mil lb) | 3.1 | 3.4 | 6.0 | 6.3 | 5.6 | 4.7 | 4.6 | 4.0 | 2.6 | 2.3 | 2.0 | 2.3 | 2.7 | 4.1 | 4.5 | 3.9 | 4.1 |
U3O8 (tonnes) | 1,410 | 1,540 | 2,700 | 2,860 | 2,540 | 2,130 | 2,090 | 1,800 | 1,180 | 1,040 | 910 | 1,040 | 1,220 | 1,860 | 2,040 | 1,770 | 1,860 |
Uranium mining declined with the last open pit mine shutting down in 1992 (Shirley Basin, Wyoming). United States production occurred in the following states (in descending order): New Mexico, Wyoming, Colorado, Utah, Texas, Arizona, Florida, Washington, and South Dakota. The collapse of uranium prices caused all conventional mining to cease by 1992. "In-situ" recovery or ISR has continued primarily in Wyoming and adjacent Nebraska as well has recently restarted in Texas.
- Canada – 1959, 2001?: The first phase of Canadian uranium production peaked at more than 12 kilotonnes (26×10 6 lb) in 1959.[137] The 1970s saw renewed interest in exploration and resulted in major discoveries in northern Saskatchewan's Athabasca Basin. Production peaked its uranium production a second time at 12,522 tonnes (28×10 6 lb) in 2001. Experts believe that it will take more than ten years to open new mines.[56]
World peak uranium
Historical opinions of world uranium supply limits
In 1943, Alvin M. Weinberg et al. believed that there were serious limitations on nuclear energy if only U-235 were used as a nuclear power plant fuel.[138] They concluded that breeding was required to usher in the age of nearly endless energy.
In 1956, M. King Hubbert declared world fissionable reserves adequate for at least the next few centuries, assuming breeding and reprocessing would be developed into economical processes.[5]
In 1975 the US Department of the Interior, Geological Survey, distributed the press release "Known US Uranium Reserves Won't Meet Demand". It was recommended that the US not depend on foreign imports of uranium.[138]
Pessimistic predictions
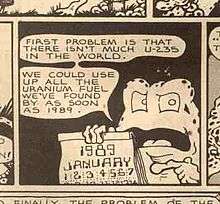
All the following sources predict peak uranium:
- Edward Steidle
Edward Steidle, Dean of the School of Mineral Industries at Pennsylvania State College, predicted in 1952 that supplies of fissionable elements were too small to support commercial-scale energy production.[140]
- 1980 Robert Vance
Robert Vance,[141] while looking back at 40 years of uranium production through all of the Red Books, found that peak global production was achieved in 1980 at 69,683 tonnes (150×10 6 lb) from 22 countries.[43] In 2003, uranium production totaled 35,600 tonnes (78×10 6 lb) from 19 countries.
- 1981 Michael Meacher
Michael Meacher, the former environment minister of the UK 1997–2003, and UK Member of Parliament, reports that peak uranium happened in 1981. He also predicts a major shortage of uranium sooner than 2013 accompanied with hoarding and its value pushed up to the levels of precious metals.[142]
- 1989–2015 M. C. Day
Day projected that uranium reserves could run out as soon as 1989, but, more optimistically, would be exhausted by 2015.[139]
- 2034 van Leeuwen
Jan Willem Storm van Leeuwen, an independent analyst with Ceedata Consulting, contends that supplies of the high-grade uranium ore required to fuel nuclear power generation will, at current levels of consumption, last to about 2034.[143] Afterwards, the cost of energy to extract the uranium will exceed the price the electric power provided.
- 2035 Energy Watch Group
The Energy Watch Group has calculated that, even with steep uranium prices, uranium production will have reached its peak by 2035 and that it will only be possible to satisfy the fuel demand of nuclear plants until then.[144]
Various agencies have tried to estimate how long these resources will last.
- European Commission
The European Commission said in 2001 that at the current level of uranium consumption, known uranium resources would last 42 years. When added to military and secondary sources, the resources could be stretched to 72 years. Yet this rate of usage assumes that nuclear power continues to provide only a fraction of the world’s energy supply. If electric capacity were increased six-fold, then the 72-year supply would last just 12 years.[56]
- OECD
The world's present measured resources of uranium, economically recoverable at a price of US$130/kg according to the industry groups OECD, NEA and IAEA, are enough to last for 100 years at current consumption.[8]
- Australian Uranium Association
According to the Australian Uranium Association, yet another industry group, assuming the world's current rate of consumption at 66,500 tonnes of uranium per year and the world's present measured resources of uranium (4.7 Mt) are enough to last for 70 years.[58]
Optimistic predictions
All the following references claim that the supply is far more than demand. Therefore, they do not predict peak uranium.
- M. King Hubbert
In his 1956 landmark paper, M. King Hubbert wrote "There is promise, however, provided mankind can solve its international problems and not destroy itself with nuclear weapons, and provided world population (which is now expanding at such a rate as to double in less than a century) can somehow be brought under control, that we may at last have found an energy supply adequate for our needs for at least the next few centuries of the 'foreseeable future.'"[5] Hubbert's study assumed that breeder reactors would replace light water reactors and that uranium would be bred into plutonium (and possibly thorium would be bred into uranium). He also assumed that economic means of reprocessing would be discovered. For political, economic and nuclear proliferation reasons, the plutonium economy never materialized. Without it, uranium is used up in a once-through process and will peak and run out much sooner.[145] However, at present, it is generally found to be cheaper to mine new uranium out of the ground than to use reprocessed uranium, and therefore the use of reprocessed uranium is limited to only a few nations.
- OECD
The OECD estimates that with the world nuclear electricity generating rates of 2002, with LWR, once-through fuel cycle, there are enough conventional resources to last 85 years using known resources and 270 years using known and as yet undiscovered resources. With breeders, this is extended to 8,500 years.[146]
If one is willing to pay $300/kg for uranium, there is a vast quantity available in the ocean.[8] It is worth noting that since fuel cost only amounts to a small fraction of nuclear energy total cost per kWh, and raw uranium price also constitutes a small fraction of total fuel costs, such an increase on uranium prices wouldn’t involve a very significant increase in the total cost per kWh produced.
- Bernard Cohen
In 1983, physicist Bernard Cohen proposed that uranium is effectively inexhaustible, and could therefore be considered a renewable source of energy.[6] He claims that fast breeder reactors, fueled by naturally replenished uranium extracted from seawater, could supply energy at least as long as the sun's expected remaining lifespan of five billion years.[6] While uranium is a finite mineral resource within the earth, the hydrogen in the sun is finite too – thus, if the resource of nuclear fuel can last over such time scales, as Cohen contends, then nuclear energy is every bit as sustainable as solar power or any other source of energy, in terms of sustainability over the time scale of life surviving on this planet.
We thus conclude that all the world’s energy requirements for the remaining 5×109 yr of existence of life on Earth could be provided by breeder reactors without the cost of electricity rising by as much as 1% due to fuel costs. This is consistent with the definition of a "renewable" energy source in the sense in which that term is generally used.
His paper assumes extraction of uranium from seawater at the rate of 16 kilotonnes (35×10 6 lb) per year of uranium.[6] The current demand for uranium is near 70 kilotonnes (150×10 6 lb) per year; however, the use of breeder reactors means that uranium would be used at least 60 times more efficiently than today.
- James Hopf
A nuclear engineer writing for American Energy Independence in 2004 believes that there is a several hundred years' supply of recoverable uranium even for standard reactors. For breeder reactors, "it is essentially infinite".[147] All the following references claim that the supply is far more than demand. Therefore, they believe that uranium will not deplete in the foreseeable future.
- IAEA
The IAEA estimates that using only known reserves at the current rate of demand and assuming a once-through nuclear cycle that there is enough uranium for at least 100 years. However, if all primary known reserves, secondary reserves, undiscovered and unconventional sources of uranium are used, uranium will be depleted in 47,000 years.[8]
- Kenneth S. Deffeyes
Kenneth S. Deffeyes estimates that if one can accept ore one tenth as rich then the supply of available uranium increased 300 times.[41][148] His paper shows that uranium concentration in ores is log-normal distributed. There is relatively little high-grade uranium and a large supply of very low grade uranium.
Ernest J. Moniz, a professor at the Massachusetts Institute of Technology and the current United States Secretary of Energy, testified in 2009 that an abundance of uranium had put into question plans to reprocess spent nuclear fuel. The reprocessing plans dated from decades previous, when uranium was thought to be scarce. But now, "roughly speaking, we’ve got uranium coming out of our ears, for a long, long time," Professor Moniz said.[149]
Possible effects and consequences
As uranium production declines, uranium prices would be expected to increase. However, the price of uranium makes up only 9% of the cost of running a nuclear power plant, much lower than the cost of coal in a coal-fired power plant (77%), or the cost of natural gas in a gas-fired power plant (93%).[150][151]
Uranium is different from conventional energy resources, such as oil and coal, in several key aspects. Those differences limit the effects of short-term uranium shortages, but most have no bearing on the eventual depletion. Some key features are:
- The uranium market is diverse, and no country has a monopoly influence on its prices.
- Thanks to the extremely high energy density of uranium, stockpiling of several years' worth of fuel is feasible.
- Significant secondary supplies of already mined uranium exist, including decommissioned nuclear weapons, depleted uranium tails suitable for reenrichment, and existing stockpiles.
- Vast amounts of uranium, roughly 800 times the known reserves of mined uranium, are contained in extremely dilute concentrations in seawater.
- Introduction of fast neutron reactors, combined with seawater uranium extraction, would make the uranium supply virtually inexhaustible.[152] There are currently seven experimental fast neutron reactors running globally, in India, Japan, Russia and China.[153]
Fast neutron reactors (breeder reactors) could utilize large amounts of Uranium-238 indirectly by conversion to Plutonium-239, rather than fissioning primarily just Uranium-235 (which is 0.7% of original mined uranium), for approximately a factor of 100 increase in uranium usage efficiency.[152] Intermediate between conventional estimates of reserves and the 40 trillion tons total of uranium in Earth's crust (trace concentrations adding up over its 3 * 1019 ton mass), there are ores of lower grade than otherwise practical but of still higher concentration than the average rock.[37][38] Accordingly, resource figures depend on economic and technological assumptions.
Uranium price

The uranium spot price has increased from a low in Jan 2001 of US$6.40 per pound of U3O8 to a peak in June 2007 of US$135. The uranium prices have dropped substantially since.[154] Currently (15 July 2013) the uranium spot is US$38.[155]
The high price in 2007 resulted from shrinking weapons stockpiles and a flood at the Cigar Lake Mine, coupled with expected rises in demand due to more reactors coming online, leading to a uranium price bubble. Miners and Utilities are bitterly divided on uranium prices.[156]
As prices go up, production responds from existing mines, and production from newer, harder to develop or lower quality uranium ores begins. Currently, much of the new production is coming from Kazakhstan. Production expansion is expected in Canada and in the United States. However, the number of projects waiting in the wings to be brought online now are far less than there were in the 1970s. There have been some encouraging signs that production from existing or planned mines is responding or will respond to higher prices. The supply of uranium has recently become very inelastic. As the demand increases, the prices respond dramatically.
Number of contracts
Unlike other metals such as gold, silver, copper or nickel, uranium is not widely traded on an organized commodity exchange such as the London Metal Exchange. It is traded on the NYMEX but on very low volume.[157] Instead, it is traded in most cases through contracts negotiated directly between a buyer and a seller.[158] The structure of uranium supply contracts varies widely. The prices are either fixed or base on referenced to economic indices such as GDP, inflation or currency exchange. Contracts traditionally are based on the uranium spot price and rules by which the price can escalate. Delivery quantities, schedules, and prices vary from contract to contract and often from delivery to delivery within the term of a contract.
Since the number of companies mining uranium is small, the number of available contracts is also small. Supplies are running short due to flooding of two of the world's largest mines and a dwindling amount of uranium salvaged from nuclear warheads being removed from service.[159] While demand for the metal has been steady for years, the price of uranium is expected to surge as a host of new nuclear plants come online.
Mining
Rising uranium price entices draws investment into new uranium mining projects.[156] Mining companies are returning to abandoned uranium mines with new promises of hundreds of jobs and millions in royalties. Some locals want them back. Others say the risk is too great, and will try to stop those companies "until there's a cure for cancer."[160]
Electric utilities
Since many utilities have extensive stockpiles and can plan many months in advance, they take a wait-and-see approach on higher uranium costs. In 2007, spot prices rose significantly due to announcements of planned reactors or new reactors coming online.[161] Those trying to find uranium in a rising cost climate are forced to face the reality of a seller’s market. Sellers remain reluctant to sell significant quantities. By waiting longer, sellers expect to get a higher price for the material they hold. Utilities on the other hand, are very eager to lock up long-term uranium contracts.[156]
According to the NEA, the nature of nuclear generating costs allows for significant increases in the costs of uranium before the costs of generating electricity significantly increase. A 100% increase in uranium costs would only result in a 5% increase in electric cost.[59] This is because uranium has to be converted to gas, enriched, converted back to yellow cake and fabricated into fuel elements. The cost of the finished fuel assemblies are dominated by the processing costs, not the cost of the raw materials.[162] Furthermore, the cost of electricity from a nuclear power plant is dominated by the high capital and operating costs, not the cost of the fuel. Nevertheless, any increase in the price of uranium is eventually passed on to the consumer either directly or through a fuel surcharge.
Substitutes
An alternative to uranium is thorium which is three times more common than uranium. Fast breeder reactors are not needed. Compared to conventional uranium reactors, thorium reactors using the thorium fuel cycle may produce some 40 times the amount of energy per unit of mass.[163]
If nuclear power prices rise too quickly, or too high, power companies may look for substitutes in fossil energy (coal, oil, and gas) and/or renewable energy, such as hydro, bio-energy, solar thermal electricity, geothermal, wind, tidal energy. Both fossil energy and some renewable electricity sources (e.g. hydro, bioenergy, solar thermal electricity and geothermal) can be used as base-load.
See also
References
- 1 2 "Key Characteristics of Nonrenewable Resources". API. 2006-08-24. Retrieved 2008-04-18.
- ↑ "Coal Equivalent". Retrieved 2013-06-01.
- ↑ "Non-renewable energy". DOE. Retrieved 2008-05-09.
- ↑ "Facts from Cohen".
- 1 2 3 4 M. King Hubbert (June 1956). "Nuclear Energy and the Fossil Fuels 'Drilling and Production Practice'" (PDF). API. p. 36. Retrieved 2008-04-18.
- 1 2 3 4 5 6 Cohen, Bernard L. (January 1983). "Breeder reactors: A renewable energy source" (PDF). American Journal of Physics. 51 (1): 75–6. Bibcode:1983AmJPh..51...75C. doi:10.1119/1.13440. Retrieved 2007-08-03.
- ↑ "Statement of Dr. Phillip J. Finck, Before the House Committee on Science, Energy Subcommittee Hearing on Nuclear Fuel Reprocessing". Argonne National Laboratory. 2005-06-16. Archived from the original on May 9, 2008. Retrieved 2008-05-14.
- 1 2 3 4 5 6 7 8 NEA, IAEA (2014). Uranium 2014 – Resources, Production and Demand (PDF). OECD Publishing. doi:10.1787/uranium-2014-en. ISBN 978-92-64-22351-6.
- ↑ http://large.stanford.edu/courses/2012/ph241/ferguson2/. Missing or empty
|title=
(help) - 1 2 3 Steve Fetter & Frank N. von Hippel (September 2005). "Is U.S. Reprocessing Worth The Risk?". Arms Control Association. Retrieved 2004-04-23.
- ↑ Joseph D. Parent & J. Glenn Seay (1978). "A Survey of United States and Total World Production, Proved Reserves, and Remaining Recoverable Resources of Fossil Fuels and Uranium as of December 31, 1976" (PDF). Henry R. Linden Gas Research Institute. Retrieved 2008-05-06.
- ↑ "Buyers' market definition". businessdictionary.com. Retrieved 2008-04-28.
- ↑ "Seller's market definition". businessdictionary.com. Retrieved 2008-04-28.
- 1 2 3 4 "Uranium Resources and Nuclear Energy" (PDF). Energy Watch Group. December 2006. Retrieved 2012-04-07.
- 1 2 3 "Uranium Resources 2003: Resources, Production and Demand" (PDF). OECD World Nuclear Agency and International Atomic Energy Agency. March 2008. p. 29. Retrieved 2008-04-23.
- ↑ "World Consumption of Primary Energy by Energy Type and Selected Country Groups, 1980–2004" (XLS). Energy Information Administration, U.S. Department of Energy. July 31, 2006. Retrieved 2007-01-20.
- ↑ "WNN World Nuclear News". World Nuclear Association. Retrieved January 2009. Check date values in:
|access-date=
(help) - ↑ "Global Uranium Resources to Meet Projected Demand Latest Edition of "Red Book" Predicts Consistent Supply Up to 2025". IAEA. 2006-06-02. Retrieved 2008-04-18.
- 1 2 Steve Kidd (1 September 2016). "Uranium - the market, lower prices and production costs". Nuclear Engineering International. Retrieved 19 September 2016.
- ↑ "Uranium 101 – Markets". Cameco Corporation. 2007-04-09. Retrieved 2008-05-01.
- 1 2 John Busby (2005-10-31). "Why nuclear power is not a sustainable source of low carbon energy". Hubbert Peak. Retrieved 2008-04-18.
- ↑ "The World Factbook". CIA. 2012.
- ↑ "World Population Ageing: 1950–2050". UN. 2002.
- ↑ "Map No. 11 – Population 2050". Worldmapper.org. 2005. Retrieved 2008-04-18.
- ↑ Wang Ying & Winnie Zhu (2008-06-11). "China Plans More Nuclear Reactors, Uranium Imports". Bloomberg. Retrieved 2008-10-28.
- ↑ "Nuclear Power in India". World Nuclear Association. October 2008. Retrieved 2008-10-28.
- ↑ David McKay (2006-04-26). "Uranium price tipped to reach $100/lb". MiningMX.com. Retrieved 2008-03-15.
- ↑ "Environmentalists rethink stance on nuclear power". National Public Radio. 2008-04-25. Retrieved 2008-03-28.
- ↑ "Greenhouse Emissions of Nuclear Power". nuclearinfo.net. Retrieved 2009-03-11.
- ↑ Tom Doggett (2008-02-01). "U.S. nuclear power plants to get more Russia uranium". Reuters.
- 1 2 3 "What is uranium? How does it work?". World Nuclear Association. June 2006. Retrieved 2009-10-25.
- 1 2 http://gabe.web.psi.ch/pdfs/Critical%20note%20GHG%20PSI.pdf Dones, Roberto, 2007. Critical note on the estimation by storm van Leeuwen J.W. and Smith P. of the energy uses and corresponding CO2 emissions from the complete nuclear energy chain. Paul Scherrer Institute Policy Report, April
- ↑ "i05".
- ↑ http://web.mit.edu/nuclearpower/pdf/nuclearpower-update2009.pdf p. 12
- 1 2 "Uranium Supplies: Supply of Uranium - World Nuclear Association".
- ↑ "About Uranium". Axton. Retrieved 2008-06-21.
- 1 2 Sevior M. (2006). "Considerations for nuclear power in Australia" (PDF). International Journal of Environmental Studies. 63 (6): 859–72. doi:10.1080/00207230601047255.
- 1 2 American Geophysical Union, Fall Meeting 2007, abstract #V33A-1161. Mass and Composition of the Continental Crust
- ↑ "Supply of Uranium". World Nuclear Association. June 2008. Retrieved 2008-06-21.
- 1 2 3 "Uranium Resources 2003: Resources, Production and Demand" (PDF). OECD World Nuclear Agency and International Atomic Energy Agency. March 2008. p. 22. Retrieved 2008-04-23.
- 1 2 Deffeyes, K.S.; MacGregor, I.D. (1980-01-01). "Citation for World uranium resources". 242 (1). Scientific American: 50–60. Retrieved 2008-04-26.
- 1 2 3 "UxC: World Uranium Production". UxC Consulting Company, LLC. 2007-11-27. Retrieved 2008-05-01.
- 1 2 Robert Vance. "What can 40 Years of Red Books Tell Us?". World Nuclear Association.
- ↑ Jan Slezak, "Red Book – Uranium: Resources, Production and Demand,", International Atomic Energy Agency workshop, Ghana, July 2010, p. 24.
- 1 2 "World Uranium Production". UxC. 2007-11-27. Retrieved 2008-03-15.
- ↑ "World Uranium Mining, Nuclear Issues Briefing Paper 41". Australian Uranium Association. July 2007. Archived from the original on 2008-03-03. Retrieved 2008-04-15.
- ↑ "Markets". Cameco Corporation.
- 1 2 3 Michael Meacher (2006-06-07). "On the road to ruin". London: The Guardian.
- ↑ "The largest producing uranium mines in 2006" (PDF). Compass Resources NL. 2007-08-09. p. 9 of 25. Retrieved 2008-05-04.
- ↑ "Uranium production figures, 1998–2006". World Nuclear Association. May 2007. Retrieved 2008-05-06.
- ↑ "Cigar Lake floods again". Nuclear Engineering International. August 22, 2008. Retrieved 2009-02-03.
- ↑ "Nuclear power companies hunker down as uranium prices soar". MarketWatch. 2007-03-30.
- ↑ Charles Q. Choi (2008-04-22). "Uranium Supply Decline Clouds Nuclear Power's Future". LiveScience. Retrieved 2008-05-01.
- 1 2 "Uranium reserves". European Nuclear Society. Retrieved 2008-05-09.
- ↑ "World Uranium Mining, Nuclear Issues Briefing Paper 41". Australian Uranium Association. July 2007. Archived from the original on 2008-03-03. Retrieved 2008-04-15.
- 1 2 3 Uranium shortage poses threat (2005-08-15). "Uranium shortage poses threat". London: The Times. Retrieved 2008-04-25.
- 1 2 "Uranium resources sufficient to meet projected nuclear energy requirements long into the future". Nuclear Energy Agency (NEA). 3 June 2008. Retrieved 2008-06-16.
Uranium 2007: Resources, Production and Demand, also known as the Red Book, estimates the identified amount of conventional uranium resources which can be mined for less than USD 130/kg to be about 5.5 million tonnes, up from the 4.7 million tonnes reported in 2005. Undiscovered resources, i.e. uranium deposits that can be expected to be found based on the geological characteristics of already discovered resources, have also risen to 10.5 million tonnes. This is an increase of 0.5 million tonnes compared to the previous edition of the report. The increases are due to both new discoveries and re-evaluations of known resources, encouraged by higher prices.
- 1 2 3 "Supply of Uranium". World Nuclear Association. September 2009.
- 1 2 3 4 R. Price; J.R. Blaise (2002). "Nuclear fuel resources: Enough to last?." (PDF). NEA News No. 20.2, Issy-les-Moulineaux, France.
- ↑ "Supply of Uranium". World Nuclear Association. March 2007. Retrieved 2008-05-14.
- ↑ Hisane Masaki (2006-04-22). "Japan Joins the Race for Uranium Amid Global Expansion of Nuclear Power". The Asia-Pacific Journal: Japan Focus. Retrieved 2009-03-23.
- ↑ "Uranium Resources 2003: Resources, Production and Demand" (PDF). OECD World Nuclear Agency and International Atomic Energy Agency. March 2008. p. 20. Retrieved 2008-04-23.
- ↑ "Terrestrial Gamma Radioactivity". USGS. Retrieved 2008-04-25.
- ↑ "Statement of Dr. Suzanne D. Weedman, Energy Resources Program Coordinator, USGS, U.S. Dept of the Interior before the Energy Subcommittee of the Science Committee, U.S. House of Representatives". U.S. Department of the Interior. 2001-05-03. Retrieved 2008-10-28.
- ↑ Colin MacDonald (2003). "Uranium:Sustainable Resource or Limit to Growth?". World Nuclear Association.
- ↑ "Markets". Cameco Corporation.
- ↑ "Total Commercial Uranium Inventories of U.S. Suppliers and Owners and Operators of U.S. Civilian Nuclear Power Reactors". DOE. 2007-05-16. Retrieved 2008-05-03.
- ↑ "Total Commercial Uranium Inventories of U.S. Suppliers and Owners and Operators of U.S. Civilian Nuclear Power Reactors". DOE. 2007-05-18. Retrieved 2008-05-03.
- ↑ Linda Gunter (January 2006). "Uranium Inventories" (PDF). United States Department of Energy (DOE).
- 1 2 "Megatons to Megawatts". USEC. Archived from the original on July 16, 2008. Retrieved 2008-06-11.
- ↑ "Military Warheads as a Source of Nuclear Fuel". World Nuclear Association. January 2009.
- ↑ "Military Warheads as a Source of Nuclear Fuel – Nuclear Issues Briefing Paper". World Nuclear Association. January 2009.
- ↑ "Detailed Information on the National Nuclear Security Administration: Fissile Materials Disposition Program Assessment". US White House. 2006. Retrieved 2008-05-15.
- ↑ "Reprocessing plants, world-wide". European Nuclear Society. Retrieved 2008-05-14.
- ↑ "Reprocessing plants, world-wide". European Nuclear Society. Retrieved 2008-07-29.
- ↑ Carolyn Krause (2008). "The Missing Piece – ORNL seeks to close the nuclear fuel cycle.". 41 (1). ORNL. Retrieved 2008-03-15.
- ↑ Carson Mark; Theodore Taylor; Eugene Eyster; William Maraman; Jacob Wechsler. "Can Terrorists Build Nuclear Weapons?". Nuclear Control Institute. Retrieved 2008-10-28.
- ↑ "Uranium and Depleted Uranium - Nuclear Issues Briefing Paper". World Nuclear Association. January 2009.
- 1 2 "Nuclear Reprocessing: Dangerous, Dirty, and Expensive". Union of Concerned Scientists. January 2006.
- ↑ Mary Bird Davis. "Nuclear France – Materials and Science". La France nucléaire.
- ↑ Matthew Bunn; Bob van der Zwaan; John P. Holdren & Steve Fetter (2003). "The Economics of Reprocessing vs. Direct Disposal of Spent Nuclear Fuel". Harvard University. Retrieved 2009-03-23.
- 1 2 3 4 "Survey of Energy Resources 2007 Uranium - Resources". World Energy Council. 2007.
- ↑ Ted Jackovics (2007-05-11). "Phosphate industry may restart uranium mining as price soars". Herald Tribune.
- ↑ "Analysis of Uranium Supply to 2050 – STI-PUB-1104" (PDF). IAEA. May 2001. Retrieved 2008-05-07.
- ↑ "Uranium Recovery from Phosphates". Wise Uranium Project. 2008-02-17. Retrieved 2008-05-07.
- ↑ US EIA, Uranium marketing, Table 1, accessed 3 Oct. 2013.
- ↑ "Survey of Energy Resources 2007: Uranium Resources". World Energy Council. 2007. Retrieved 2008-05-14.
- ↑ Noriaki Seko; Akio Katakai; Shin Hasegawa; Masao Tamada; Noboru Kasai; Hayato Takeda; Takanobu Sugo; Kyoichi Saito (November 2003). "Aquaculture of Uranium in Seawater by a Fabric-Adsorbent Submerged System". Nuclear Technology. American Nuclear Society. 144 (2). Retrieved 2008-04-30.
- ↑ Tamada M, et al. (2006). "Cost Estimation of Uranium Recovery from Seawater with System of Braid type Adsorbent". 5 (4). Nippon Genshiryoku Gakkai Wabun Ronbunshi.: 358–63. Retrieved 2008-05-02.
- ↑ http://www.ornl.gov/info/press_releases/get_press_release.cfm?ReleaseNumber=mr20120821-00
- ↑ "PNNL: News - Fueling nuclear power with seawater".
- ↑ "Nanofibers Extract Uranium from Seawater Hidden within the oceans, scientists have found a possible way to power nuclear reactors long after uranium mines dry up.".
- ↑ "abstracts from papers for the ACS Extraction of Uranium from Seawater conference".
- ↑ "Advances in decades-old dream of mining seawater for uranium".
- ↑ E. A. Heide, K. Wagener, M. Paschke and M. Wald (September 1973). "Extraction of uranium from sea water by cultured algae". 60 (9). Retrieved 2008-04-22.
- ↑ Cooper, Christopher, H.; et al. (2003-03-07). "Purification of fluids with nanomaterials". Retrieved 2008-04-22.
- ↑ U.S. Geological Survey (October 1997). "Radioactive Elements in Coal and Fly Ash: Abundance, Forms, and Environmental Significance" (PDF). U.S. Geological Survey Fact Sheet FS-163-97.
- 1 2 Coal Combustion – ORNL Review Vol. 26, No. 3&4, 1993
- ↑ "Factsheets & FAQs". International Atomic Energy Agency (IAEA). Retrieved 2012-02-01.
- ↑ US Energy Information Administration, Belfield Ashing facility site.
- 1 2 "Sparton produces first yellowcake from Chinese coal ash" (PDF). World Nuclear News. October 2007. Retrieved 2008-05-14.
- ↑ Dyni, John R. (2006). "Geology and resources of some world oil-shale deposits. Scientific Investigations Report 2005–5294" (PDF). U.S. Department of the Interior. U.S. Geological Survey. Retrieved 2007-07-09.
- ↑ "Fast Reactor Technology: A Path to Long-Term Energy Sustainability" (PDF). American Nuclear Society. November 2005. Retrieved 2008-05-14.
- ↑ McCarthy, J. (12 February 1996). "Facts from Cohen and others". Progress and its Sustainability. Stanford University. Retrieved 2007-08-03.
- ↑ Clark, Duncan (2012-07-09). "Nuclear waste-burning reactor moves a step closer to reality". The Guardian. London.
- 1 2 "Fast Neutron Reactors". World Nuclear Association. February 2008. Retrieved 2008-05-13.
- ↑ Henry Sokolski (1982-09-24). "Clinch River: The SST of the Eighties". The Heritage Foundation.
- ↑ Arjun Makhijani. "Plutonium End Game: Stop Reprocessing, Start Immobilizing". IEER. Retrieved 2008-04-28.
- ↑ "Research Note 01/03 – Dounreay" (PDF). The Scottish Parliament – The information centre. 2001-01-09. Archived from the original (PDF) on September 24, 2004. Retrieved 2008-04-28.
- ↑ "Fast Neutron Reactors". World Nuclear Association. November 2007.
- ↑ Keiko Chino & Yomiuri Shimbun (2008-10-25). "N-power debate at crossroads / Falling oil prices, poor research results cast doubt on industry's future". Yomiuri Shimbun. Retrieved 2008-10-28.
- ↑ "Generation IV International Forum Signs Agreement to Collaborate on Sodium Cooled Fast Reactors". US Department of Energy. 2006-02-17. Retrieved 2009-03-12.
- ↑ "Nuclear Power in France".
- ↑ "France, Japan, US cooperate on nuclear reactors". Space Daily. 2008-02-01.
- ↑ "Thorium". Australian Uranium Association / World Nuclear Association. January 2009.
- ↑ Mujid S. Kazimi (September–October 2003). "Thorium Fuel for Nuclear Energy - Now You're Cooking with Thorium". 91 (5). American Scientist: 408. Archived from the original on January 2, 2008.
- ↑ "India to build prototype thorium reactor". bellona. 2003-09-25.
- ↑ "Use of Thorium reserve depends on Uranium". The Indian Express. 2008-06-19. Retrieved 2008-10-29.
- ↑ Martin, Richard (2011-02-01), "China Takes Lead in Race for Clean Nuclear Power", Wired Science
- ↑ http://whb.news365.com.cn/yw/201101/t20110126_2944856.htm
- ↑ "中国科学院2011年度工作会议在京召开----中国科学院".
- ↑ Clark, Duncan (16 February 2011). "China enters race to develop nuclear energy from thorium". The Guardian. London.
- ↑ "China's Nuclear Fuel Cycle". World Nuclear Association. April 2012.
- ↑ Uranium 2003, OCED Nuclear Energy Agency, and International Atomic Energy Agency, 2004.
- ↑ "Uranium resources: plenty to sustain growth of nuclear power". Nuclear Energy Agency. 2006-06-01. Retrieved 2008-04-26.
- ↑ "World Nuclear Power Reactors 2006-08 and Uranium Requirements". World Nuclear Association. 2008-01-14. Retrieved 2008-04-26.
- 1 2 "World Uranium Production U3O8/ million lbs". Ux Consulting Company, LLC. 2007-11-07. Retrieved 2008-04-26.
- ↑ Taryn Toro (1991-06-22). "How to close a uranium mine". New Scientist.
- ↑ Steve Christ (2006-12-01). "India's Peak Uranium Problem Invites New Conquerors India's Peak Uranium Problem Invites New Conquerors". Energy and Capital. Retrieved 2008-02-06.
- ↑ "The Ranstad Uranium Mine in Sweden".
- ↑ Peter Diehl (September 1995). "Uranium production in Europe - The Impacts on Man and Environment".
- ↑ Winfried Koelzer (1999). "Uranium mining, global". European Nuclear Society.
- ↑ "Uranium Resources 2003: Resources, Production and Demand" (PDF). OECD World Nuclear Agency and International Atomic Energy Agency. March 2008. p. 237. Retrieved 2008-04-23.
- ↑ Bureau, Commodity Research; Inc, (2004). The CRB Commodity Yearbook 2004 - Uranium, Supply. Commodity Research Bureau, Inc. p. 293. ISBN 978-0-471-64921-2. Retrieved 2008-04-24.
- ↑ "Domestic Uranium Production Report - 2006 Summary". DOE-Energy Information Administration. 2007-05-04.
- ↑ "Summary Production Statistics of the U.S. Uranium Industry". DOE. 2008-05-13. Retrieved 2008-05-16.
- ↑ "Canada's Uranium Production & Nuclear Power". World Nuclear Association. March 2003. Retrieved 2008-04-24.
- 1 2 Samuel Upton Newtan (2007). Nuclear War I and Other Major Nuclear Disasters of the 20th Century. AuthorHouse. p. 173. ISBN 978-1-4259-8510-3. Retrieved 2009-04-13.
- 1 2 Day, M. C. (1975). "Nuclear Energy: A Second Round of Questions". Bulletin of the Atomic Scientists. 31 (10): 52–9. Retrieved 13 February 2013. Note case 1 on page 57 which gives 1989 as the year by which reserves could be expended.
- ↑ Edward Steidle, 'Mineral Forecast 2000 A.D.' (State College, Penn.: Pennsylvania State College, 1952) 178.
- ↑ "Biography of Robert Vance". World Nuclear Association. 2006. Retrieved 2008-05-09.
- ↑ Michael Meacher (2006-06-07). "On the road to ruin". London: The Guardian. Retrieved 2008-05-09.
- ↑ Jan Willem Storm van Leeuwen (2006-7). "Secure energy: options for a safer world - Energy security and uranium reserves" (PDF). Oxford Research Group. Archived from the original (PDF) on 2008-11-21. Check date values in:
|date=
(help) - ↑ "Energy Watch Group warns: Depleting uranium reserves dash hopes for atomic energy supply". Sonnenseite. 2006-06-12.
- ↑ Dave Kimble. "Is there enough Uranium to run a nuclear industry big enough to take over from fossil fuels?". davekimble.org.au. Retrieved 2013-09-15.
- ↑ "Uranium Resources 2003: Resources, Production and Demand" (PDF). OECD World Nuclear Agency and International Atomic Energy Agency. March 2008. p. 65. Retrieved 2008-04-23.
- ↑ "World Uranium Reserves".
- ↑ "World Uranium Resources", by Kenneth S. Deffeyes and Ian D. MacGregor, Scientific American, January, 1980, page 66, argues that the supply of uranium is very large.
- ↑ Wald, Matthew L. (2009-09-23). "U.S. Panel Shifts Focus to Reusing Nuclear Fuel". The New York Times. Retrieved 2010-05-27.
- ↑ "Nuclear energy's future from a uranium producer's perspective", Mining Engineering, October 2008, p. 29.
- ↑ "Nuclear Economics". World Nuclear Association. January 2010. Retrieved 2010-02-21.
- 1 2 http://sustainablenuclear.org/PADs/pad11983cohen.pdf
- ↑ "Nuclear Fusion : WNA - World Nuclear Association".
- 1 2 "NUEXCO Exchange Value (Monthly Uranium Spot)".
- ↑ "UxC Nuclear Fuel Price Indicators".
- 1 2 3 James Finch & Julie Ickes (2007-06-08). "Utilities, Miners Bitterly Divided on Uranium Price Rise". StockInterview.
- ↑ url=http://www.nymex.com/UX_csf.aspx
- ↑ "Uranium 101: Markets". Cameco.
- ↑ Steve Hargreaves (2007-04-19). "What's behind the red-hot uranium boom". CNN.
- ↑ Zsombor Peter (2007-07-16). "Too hot to handle?". The Gallup Independent.
- ↑ "U.S. Utilities Quietly Worry about Uranium Supply". 2007-04-15.
- ↑ "The Economics of Nuclear Power". Australian Uranium Association / World Nuclear Association. January 2009.
- ↑ "Thorium". World Nuclear Association. March 2008. Retrieved 2008-05-14.
Further reading
- Books
- Herring, J.: Uranium and Thorium Resource Assessment, Encyclopedia of Energy, Boston University, Boston, 2004, ISBN 0-12-176480-X.
- Articles
- Deffeyes, Kenneth S., MacGregor, Ian D. "Uranium Distribution in Mined Deposits and in the Earth’s Crust" Final Report, GJBX–1(79), Dept of Geological and Geophysical Sciences, Princeton University, Princeton, NJ.
- Deffeyes, K., MacGregor, I.: "World Uranium resources" Scientific American, Vol. 242, No. 1, January 1980, pp. 66–76.