Shapiro reaction
The Shapiro reaction or tosylhydrazone decomposition is an organic reaction in which a ketone or aldehyde is converted to an alkene through an intermediate hydrazone in the presence of 2 equivalents of strong base.[1][2][3] The reaction was discovered by Robert H. Shapiro in 1967.[4] The Shapiro reaction was used in the Nicolaou Taxol total synthesis.
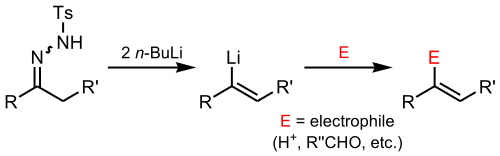
Reaction mechanism
In a prelude to the actual Shapiro reaction a ketone or an aldehyde is reacted with p-toluenesulfonylhydrazide[5] to a p-toluenesulfonylhydrazone (or tosylhydrazone) which is an imine or hydrazone. Two equivalents of a strong base, such as n-butyllithium, then abstract first the proton from the hydrazone and then the less acidic proton α to the hydrazone carbon, leaving a carbanion. The carbanion proceeds in an elimination reaction to create the carbon–carbon double bond. This step results in expulsion of the tosyl group and formation of a diazonium anion. The anion then collapses, falling off as a neutral nitrogen molecule. The result is a vinyllithium at the position where the nitrogen had been attached. This organolithium carbon is both nucleophilic and basic. It can be reacted with various electrophiles or simply neutralized with water or an acid.
Scope
The position of the alkene in the product is controlled by the site of deprotonation by the organolithium base. In general, the kinetically favored, less substituted site of differentially substituted tosylhydrazones is deprotonated selectively, leading to the less substituted vinyllithium intermediate. Although many secondary reactions exist for the vinyllithium functional group, in the Shapiro reaction in particular water is added, resulting in protonation to the alkene.[6] Other reactions of vinyllithium compounds include alkylation reactions with for instance alkyl halides.[7]

Importantly, the Shapiro reaction cannot be used to synthesize 1-lithioalkenes (and the resulting functionalized derivatives), as sulfonylhydrazones derived from aldehydes undergo exclusive addition of the organolithium base to the carbon of the C–N double bond.[8]
Catalytic Shapiro Reaction
Traditional Shapiro reactions require stoichiometric (sometimes excess) amounts of base to generate the alkenyllithium reagents. To combat this problem, Yamamoto and coworkers developed an efficient stereoselective and regioselective route to alkenes using a combination of ketone phenylaziridinylhydrazones as arenesulfonylhydrazone equivalents with a catalytic amount of lithium amides.
The required phenylaziridinylhydrazone was prepared from the condensation of 6-undecanone with 1-amino-2-phenyl-aziridine. Treatment of the phenylaziridinylhydrazone with 0.3 equivalents of LDA in ether resulted in the alkene shown below with an cis:trans ratio of 99.4:0.6. The ratio was determined by capillary GLC analysis after conversion to the corresponding epoxides with mCPBA. The catalyst loading can be reduced to 0.05 equivalents in the case of a 30 mmol scale reaction.
The high stereoselectivity is obtained by the preferential abstraction of the α-methylene hydrogen syn to the phenylaziridine, and is also accounted for by the internal chelation of the lithiated intermediated.[9]

A One Pot In Situ Combined Shapiro-Suzuki Reaction
The Shapiro reaction can also be combined with the Suzuki reaction to produce a variety of olefin products. Keay and coworkers have developed methodology that combines these reactions in a one pot process that does not require the isolation of the boronic acid, a setback of the traditional Suzuki coupling. This reaction has a wide scope, tolerating a slew of trisylhydrazones and aryl halides, as well as several solvents and Pd sources. [10]
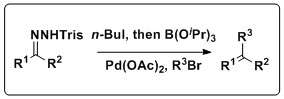
An Application of the Shapiro Reaction in Total Synthesis
The Shapiro reaction has been used to generate olefins en route to complex natural products. K. Mori and coworkers wanted to determine the absolute configuration of the phytocassane group of a class of natural products called phytoalexins. This was accomplished by preparing the naturally-occurring (–)-phytocassane D from (R)-Wieland-Miescher ketone. En route to (–)-phytocassane D, a tricyclic ketone was subjected to Shapiro reaction conditions to yield the cyclic alkene product. [11]
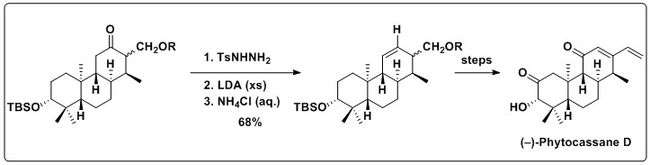
See also
References
- ↑ Shapiro, R. H.; Lipton, M.F.; Kolonko, K.J.; Buswell, R.L.; Capuano, L.A. Tetrahedron Lett., 1975, 1811. doi:10.1016/S0040-4039(00)75263-4
- ↑ Shapiro, R. H. Org. React., 1976, 23, 405. (Review)
- ↑ Adlington, R.M.; Barret, A.G.M. Acc. Chem. Res., 1983, 16, 55. (Review)
- ↑ Shapiro, Robert H.; Heath, Marsha J. J. Am. Chem. Soc., 1967, 89, 5734–5735. doi:10.1021/ja00998a601
- ↑ Organic Syntheses Coll. Vol. 5, p.1055 (1973); Vol. 40, p.93 (1960) (Article)
- ↑ Shapiro, R. H.; Duncan, J. H. Organic Syntheses Coll. Vol. 6, p.172 (1988); Vol. 51, p.66 (1971). (Article)
- ↑ Organic Syntheses Coll. Vol. 7, p.77 (1990); Vol. 61, p.141 (1983). (Article)
- ↑ Chamberlin, A. R.; Bloom, S. H. Org. React. 1990, 39, 1. doi:10.1002/0471264180.or039.01
- ↑ Yamamoto, H. (1991). "The Catalytic Shapiro Reaction". J. Am. Chem. Soc. 118: 2289-2290. doi:10.1021/ja951422p.
- ↑ Keay, B.A. (1991). "A One Pot In Situ Combined Shapiro-Suzuki Reaction". Tet. Lett. 37: 429-432.
- ↑ Yajima, A. (2000). "Diterpenoid total synthesis. XXXII. Synthesis and absolute configuration of (–)-phytocassane D, a diterpene phytoalexin isolated from the rice plant, Oryza sativa". Eur. J. Org. Chem (4079-4091).