Cyberknife
The CyberKnife is a frameless robotic radiosurgery system used for treating benign tumors, malignant tumors and other medical conditions.[1][2] The system was invented by John R. Adler, a Stanford University professor of neurosurgery and radiation oncology, and Peter and Russell Schonberg of Schonberg Research Corporation. It is made by the Accuray company headquartered in Sunnyvale, California.
The CyberKnife system is a method of delivering radiotherapy, with the intention of targeting treatment more accurately than standard radiotherapy.[3] The two main elements of the CyberKnife are:
- the radiation produced from a small linear particle accelerator (linac)
- a robotic arm which allows the energy to be directed at any part of the body from any direction

Main features
Several generations of the CyberKnife system have been developed since its initial inception in 1990. There are two major features of the CyberKnife system that are different from other stereotactic therapy methods.
Robotic mounting
The first is that the radiation source is mounted on a general purpose industrial robot. The original CyberKnife used a Japanese Fanuc robot; however, the more modern systems use a German KUKA KR 240. Mounted on the Robot is a compact X-band linac that produces 6MV X-ray radiation. The linac is capable of delivering approximately 600 cGy of radiation each minute – a new 800 cGy / minute model was announced at ASTRO[4] 2007. The radiation is collimated using fixed tungsten collimators (also referred to as "cones") which produce circular radiation fields. At present the radiation field sizes are: 5, 7.5, 10, 12.5, 15, 20, 25, 30, 35, 40, 50 and 60 mm. ASTRO 2007 also saw the launch of the IRIS[4] variable-aperture collimator which uses two offset banks of six prismatic tungsten segments to form a blurred regular dodecagon field of variable size which eliminates the need for changing the fixed collimators. Mounting the radiation source on the robot allows near-complete freedom to position the source within a space about the patient. The robotic mounting allows very fast repositioning of the source, which enables the system to deliver radiation from many different directions without the need to move both the patient and source as required by current gantry configurations.
Image guidance
The second is that the CyberKnife system uses an image guidance system. X-ray imaging cameras are located on supports around the patient allowing instantaneous X-ray images to be obtained.
6D skull
The original (and still utilized) method is called 6D or skull based tracking. The X-ray camera images are compared to a library of computer generated images of the patient anatomy. Digitally Reconstructed Radiographs (or DRR's) and a computer algorithm determines what motion corrections have to be given to the robot because of patient movement. This imaging system allows the CyberKnife to deliver radiation with an accuracy of 0.5mm without using mechanical clamps attached to the patient's skull.[5] The use of the image-guided technique is referred to as frameless stereotactic radiosurgery. This method is referred to as 6D because corrections are made for the 3 translational motions (X,Y and Z) and three rotational motions. It should be noted that it is necessary to use some anatomical or artificial feature to orient the robot to deliver X-ray radiation, since the tumor is never sufficiently well defined (if visible at all) on the X-ray camera images.
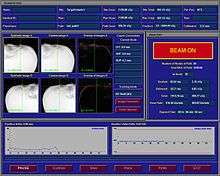
Xsight
Additional image guidance methods are available for spinal tumors and for tumors located in the lung. For a tumor located in the spine, a variant of the image guidance called Xsight-Spine[6] is used. The major difference here is that instead of taking images of the skull, images of the spinal processes are used. Whereas the skull is effectively rigid and non-deforming, the spinal vertebrae can move relative to each other, this means that image warping algorithms must be used to correct for the distortion of the X-ray camera images.
A recent enhancement to Xsight is Xsight-Lung[7] which allows tracking of some lung tumors without the need to implant fiducial markers.[8]
Fiducial
For soft tissue tumors, a method known as fiducial tracking can be utilized.[9] Small metal markers (fiducials) made out of gold for bio-compatibility and high density to give good contrast on X-ray images are surgically implanted in the patient. This is carried out by an interventional radiologist, or neurosurgeon. The placement of the fiducials is a critical step if the fiducial tracking is to be used. If the fiducials are too far from the location of the tumor, or are not sufficiently spread out from each other it will not be possible to accurately deliver the radiation. Once these markers have been placed, they are located on a CT scan and the image guidance system is programmed with their position. When X-ray camera images are taken, the location of the tumor relative to the fiducials is determined, and the radiation can be delivered to any part of the body. Thus the fiducial tracking does not require any bony anatomy to position the radiation. Fiducials are known however to migrate and this can limit the accuracy of the treatment if sufficient time is not allowed between implantation and treatment for the fiducials to stabilize.[10][11]
Synchrony
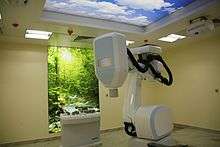
Another technology of image guidance that the CyberKnife system can use is called the Synchrony system or Synchrony method. This method uses a combination of surgically placed internal fiducials (typically small gold markers, well visible in x-ray imaging), and light emitting optical fibers (LED markers) mounted on the patient skin. LED markers are tracked by an infrared tracking camera. Since the tumor is moving continuously, to continuously image its location using X-ray cameras would require prohibitive amounts of radiation to be delivered to the patient's skin. The Synchrony system overcomes this by periodically taking images of the internal fiducials, and computing a correlation model between the motion of the external LED markers and the internal fiducials. Time stamps from the two sensors (x-ray and infrared LED) are needed to synchronize the two data streams, hence the name Synchrony.
Motion prediction is used to overcome the motion latency of the robot and the latency of image acquisition. Before treatment, a computer algorithm creates a correlation model that represents how the internal fiducial markers are moving compared to the external markers. During treatment, the system continuously infers the motion of the internal fiducials, and therefore the tumor, based on the motion of the skin markers. The correlation model is updated at fixed time steps during treatment. Thus, the Synchrony tracking method makes no assumptions about the regularity or reproducibility of the patient breathing pattern.
To function properly, the system requires that for any given correlation model there is a functional relationship between the markers and the internal fiducials. The external marker placement is also important, and the markers are usually placed on the patient abdomen so that their motion will reflect the internal motion of the diaphragm and the lungs. This method was invented in 1998.[12][13] The first patients were treated at Cleveland Clinic in 2002. Synchrony is utilized primarily for tumors that are in motion while being treated, such as lung tumors and pancreatic tumors.[14][15]
RoboCouch
A robotic six degree of freedom patient treatment couch called RoboCouch[16] improves patient positioning options for treatment.
Frameless
The frameless nature of the CyberKnife also increases the clinical efficiency. In conventional frame-based radiosurgery, the accuracy of treatment delivery is determined solely by connecting a rigid frame to the patient which is anchored to the patient’s skull with invasive aluminum or titanium screws. The CyberKnife is the only radiosurgery device that does not require such a frame for precise targeting.[17] Once the frame is connected, the relative position of the patient anatomy must be determined by making a CT or MRI scan. After the CT or MRI scan has been made, a radiation oncologist must plan the delivery of the radiation using a dedicated computer program, after which the treatment can be delivered, and the frame removed. The use of the frame therefore requires a linear sequence of events that must be carried out sequentially before another patient can be treated. Staged CyberKnife radiosurgery is of particular benefit to patients who have previously received large doses of conventional radiation therapy and patients with gliomas located near critical areas of the brain. Unlike whole brain radiotherapy, which must be administered daily over several weeks, radiosurgery treatment can usually be completed in 1–5 treatment sessions. Radiosurgery can be used alone to treat brain metastases, or in conjunction with surgery or whole brain radiotherapy, depending on the specific clinical circumstances.[18]
By comparison, using a frameless system, a CT scan can be carried out on any day prior to treatment that is convenient. The treatment planning can also be carried out at any time prior to treatment. During the treatment the patient need only be positioned on a treatment table and the predetermined plan delivered. This allows the clinical staff to plan many patients at the same time, devoting as much time as is necessary for complicated cases without slowing down the treatment delivery. While a patient is being treated, another clinician can be considering treatment options and plans, and another can be conducting CT scans.
In addition, very young patients (pediatric cases) or patients with fragile heads because of prior brain surgery cannot be treated using a frame based system. Also, by being frameless the CyberKnife can efficiently re-treat the same patient without repeating the preparation steps that a frame-based system would require.
The delivery of a radiation treatment over several days or even weeks (referred to as fractionation) can also be beneficial from a therapeutic point of view. Tumor cells typically have poor repair mechanisms compared to healthy tissue, so by dividing the radiation dose into fractions the healthy tissue has time to repair itself between treatments.[19] This can allow a larger dose to be delivered to the tumor compared to a single treatment.[20]
Clinical uses
Since August 2001, the CyberKnife system has FDA clearance for treatment of tumors in any location of the body.[21] Some of the tumors treated include: pancreas,[15][22] liver,[23] prostate,[24][25] spinal lesions,[26] head and neck cancers,[27] and benign tumors.[28]
None of these studies have shown any general survival benefit over conventional treatment methods. By increasing the accuracy with which treatment is delivered there is a potential for dose escalation, and potentially a subsequent increase in effectiveness, particularly in local control rates. However the studies cited are so far limited in scope, and more extensive research will need to be completed in order to show any effects on survival.[22]
In 2008 actor Patrick Swayze was among the people to be treated with CyberKnife radiosurgery.[29]
Locations
CyberKnife systems have been installed in over 150 locations,[30] including 100 hospitals in the United States.[31]
Recently – April 2014 – CyberKnife has been installed at Sir Charles Gairdner Hospital, Perth, Australia.[32]
Stanford University has treated over 2,500 patients using the Cyberknife system, and worldwide over 40,000 patients have been treated.[33]
See also
- Horsley–Clarke apparatus
- Gamma knife
- NCI-designated Cancer Centers in the United States
- Novalis radiosurgery
- Robotic surgery
References
- ↑ Radiosurgery/Cyberknife. Stanford School of Medicine
- ↑ Coste-Manière, E. et al. (1 March 2005) "Robotic Whole Body Stereotactic Radiosurgery: Clinical Advantages of the CyberKnife® Integrated System". Robotics Online.
- ↑ Plowman, Nick. How CyberKnife Works. London HCA
- 1 2 Accuray Announces Four New Products at Nation’s Leading Radiation Oncology Meeting. accuray.com. 29 October 2007
- ↑ Inoue, M.; Sato, K.; Koike, I. (2006). "2722". International Journal of Radiation Oncology*Biology*Physics. 66 (3): S611. doi:10.1016/j.ijrobp.2006.07.1138.
- ↑ Xsight Spine Tracking System. Accuray
- ↑ Xsight Lung Tracking System. Accuray
- ↑ Schweikard, A; Shiomi, H; Adler, J (2005). "Respiration tracking in radiosurgery without fiducials". The International Journal of Medical Robotics and Computer Assisted Surgery. 1 (2): 19–27. doi:10.1002/rcs.38. PMID 17518375.
- ↑ CyberKnife Radiosurgery – Fiducial Overview. sdcyberknife.com
- ↑ Fuller CD; Scarbrough TJ (2006). "Fiducial Markers in Image-guided Radiotherapy of the Prostate". US Oncological Disease. 1 (2): 75–9.
- ↑ Murphy, M. J. (2002). "Fiducial-based targeting accuracy for external-beam radiotherapy". Medical Physics. 29 (3): 334. doi:10.1118/1.1448823.
- ↑ Schweikard, A; Glosser, G; Bodduluri, M; Murphy, M. J.; Adler, J. R. (2000). "Robotic motion compensation for respiratory movement during radiosurgery". Computer Aided Surgery. 5 (4): 263–77. doi:10.1002/1097-0150(2000)5:4<263::AID-IGS5>3.0.CO;2-2. PMID 11029159.
- ↑ Schweikard, A; Shiomi, H; Adler, J (2004). "Respiration tracking in radiosurgery". Medical physics. 31 (10): 2738–41. doi:10.1118/1.1774132. PMID 15543778.
- ↑ Muacevic, Alexander et al. (9 December 2009) "Single-Session Lung Radiosurgery Using Robotic Image-Guided Real-Time Respiratory Tumor Tracking". Cureus.
- 1 2 Koong, A. C.; Le, Q. T.; Ho, A; Fong, B; Fisher, G; Cho, C; Ford, J; Poen, J; Gibbs, I. C.; Mehta, V. K.; Kee, S; Trueblood, W; Yang, G; Bastidas, J. A. (2004). "Phase I study of stereotactic radiosurgery in patients with locally advanced pancreatic cancer". International Journal of Radiation Oncology*Biology*Physics. 58 (4): 1017–21. doi:10.1016/j.ijrobp.2003.11.004. PMID 15001240.
- ↑ RoboCouch Patient Positioning System. Accuray
- ↑ "Rocky Mountain CyberKnife Center – Brain Metastases". rockymountainck.com.
- ↑ Chang, S. D.; Main, W; Martin, D. P.; Gibbs, I. C.; Heilbrun, M. P. (2003). "An analysis of the accuracy of the Cyber Knife: A robotic frameless stereotactic radiosurgical system". Neurosurgery. 52 (1): 140–6; discussion 146–7. doi:10.1227/00006123-200301000-00018. PMID 12493111.
- ↑ Radiobiology for the Radiologist Eric J. Hall Lippincott Williams & Wilkins; 5th edition (2000)
- ↑ Comparisons between Gamma Knife, Novalis by Brainlab and Cyberknife. peacehealth.org
- ↑ "Reimbursement Information." CyberKnife. Web. 10 March 2010.
- 1 2 Koong, A. C.; Christofferson, E; Le, Q. T.; Goodman, K. A.; Ho, A; Kuo, T; Ford, J. M.; Fisher, G. A.; Greco, R; Norton, J; Yang, G. P. (2005). "Phase II study to assess the efficacy of conventionally fractionated radiotherapy followed by a stereotactic radiosurgery boost in patients with locally advanced pancreatic cancer". International Journal of Radiation Oncology*Biology*Physics. 63 (2): 320–3. doi:10.1016/j.ijrobp.2005.07.002. PMID 16168826.
- ↑ Lieskovsky, Y. C.; Koong, A.; Fisher, G.; Yang, G.; Ho, A.; Nguyen, M.; Gibbs, I.; Goodman, K. (2005). "Phase I Dose Escalation Study of Cyber Knife Stereotactic Radiosurgery for Liver Malignancies". International Journal of Radiation Oncology*Biology*Physics. 63: S283. doi:10.1016/j.ijrobp.2005.07.483.
- ↑ Hara, W.; Patel, D.; Pawlicki, T.; Cotrutz, C.; Presti, J.; King, C. (2006). "2206". International Journal of Radiation Oncology*Biology*Physics. 66 (3): S324. doi:10.1016/j.ijrobp.2006.07.612.
- ↑ "Is CyberKnife Ready for Prime Time in Prostate Cancer?". WSJ. 28 November 2008
- ↑ Gerszten, P. C.; Ozhasoglu, C; Burton, S. A.; Vogel, W. J.; Atkins, B. A.; Kalnicki, S; Welch, W. C. (2004). "Cyber Knife frameless stereotactic radiosurgery for spinal lesions: Clinical experience in 125 cases". Neurosurgery. 55 (1): 89–98; discussion 98–9. doi:10.1227/01.neu.0000440704.61013.34. PMID 15214977.
- ↑ Liao, J. J.; Judson, B.; Davidson, B.; Amin, A.; Gagnon, G.; Harter, K. (2005). "Cyber Knife Fractionated Stereotactic Radiosurgery for the Treatment of Primary and Recurrent Head and Neck Cancer". International Journal of Radiation Oncology*Biology*Physics. 63: S381. doi:10.1016/j.ijrobp.2005.07.650.
- ↑ Bhatnagar, A. K.; Gerszten, P. C.; Ozhasaglu, C; Vogel, W. J.; Kalnicki, S; Welch, W. C.; Burton, S. A. (2005). "Cyber Knife Frameless Radiosurgery for the treatment of extracranial benign tumors". Technology in cancer research & treatment. 4 (5): 571–6. doi:10.1177/153303460500400511. PMID 16173828.
- ↑ Thomas, Liz (21 July 2008) "Patrick Swayze smiling again after 'miracle' response to cancer treatment". Mail Online.
- ↑ Accuray Achieves Milestone of 150th CyberKnife System Installed Worldwide. Reuters. December 2008
- ↑ "Accuray Reaches 100th U.S. CyberKnife System Installation". pressreleasepoint.com.
- ↑ CyberKnife in the Media. uwamedicalphysics.com. 1 May 2014
- ↑ Cutland, Laura (25 September 2005). "Maverick in a mind field – Silicon Valley / San Jose Business Journal:".
Further reading
- Principles and Practice of Stereotactic Radiosurgery, Lawrence Chin, MD and William Regine, MD, Editors (2008)